
- •Preface
- •Textbook Layout and Design
- •Preliminaries
- •See, Do, Teach
- •Other Conditions for Learning
- •Your Brain and Learning
- •The Method of Three Passes
- •Mathematics
- •Summary
- •Homework for Week 0
- •Summary
- •1.1: Introduction: A Bit of History and Philosophy
- •1.2: Dynamics
- •1.3: Coordinates
- •1.5: Forces
- •1.5.1: The Forces of Nature
- •1.5.2: Force Rules
- •Example 1.6.1: Spring and Mass in Static Force Equilibrium
- •1.7: Simple Motion in One Dimension
- •Example 1.7.1: A Mass Falling from Height H
- •Example 1.7.2: A Constant Force in One Dimension
- •1.7.1: Solving Problems with More Than One Object
- •Example 1.7.4: Braking for Bikes, or Just Breaking Bikes?
- •1.8: Motion in Two Dimensions
- •Example 1.8.1: Trajectory of a Cannonball
- •1.8.2: The Inclined Plane
- •Example 1.8.2: The Inclined Plane
- •1.9: Circular Motion
- •1.9.1: Tangential Velocity
- •1.9.2: Centripetal Acceleration
- •Example 1.9.1: Ball on a String
- •Example 1.9.2: Tether Ball/Conic Pendulum
- •1.9.3: Tangential Acceleration
- •Homework for Week 1
- •Summary
- •2.1: Friction
- •Example 2.1.1: Inclined Plane of Length L with Friction
- •Example 2.1.3: Find The Minimum No-Skid Braking Distance for a Car
- •Example 2.1.4: Car Rounding a Banked Curve with Friction
- •2.2: Drag Forces
- •2.2.1: Stokes, or Laminar Drag
- •2.2.2: Rayleigh, or Turbulent Drag
- •2.2.3: Terminal velocity
- •Example 2.2.1: Falling From a Plane and Surviving
- •2.2.4: Advanced: Solution to Equations of Motion for Turbulent Drag
- •Example 2.2.3: Dropping the Ram
- •2.3.1: Time
- •2.3.2: Space
- •2.4.1: Identifying Inertial Frames
- •Example 2.4.1: Weight in an Elevator
- •Example 2.4.2: Pendulum in a Boxcar
- •2.4.2: Advanced: General Relativity and Accelerating Frames
- •2.5: Just For Fun: Hurricanes
- •Homework for Week 2
- •Week 3: Work and Energy
- •Summary
- •3.1: Work and Kinetic Energy
- •3.1.1: Units of Work and Energy
- •3.1.2: Kinetic Energy
- •3.2: The Work-Kinetic Energy Theorem
- •3.2.1: Derivation I: Rectangle Approximation Summation
- •3.2.2: Derivation II: Calculus-y (Chain Rule) Derivation
- •Example 3.2.1: Pulling a Block
- •Example 3.2.2: Range of a Spring Gun
- •3.3: Conservative Forces: Potential Energy
- •3.3.1: Force from Potential Energy
- •3.3.2: Potential Energy Function for Near-Earth Gravity
- •3.3.3: Springs
- •3.4: Conservation of Mechanical Energy
- •3.4.1: Force, Potential Energy, and Total Mechanical Energy
- •Example 3.4.1: Falling Ball Reprise
- •Example 3.4.2: Block Sliding Down Frictionless Incline Reprise
- •Example 3.4.3: A Simple Pendulum
- •Example 3.4.4: Looping the Loop
- •3.5: Generalized Work-Mechanical Energy Theorem
- •Example 3.5.1: Block Sliding Down a Rough Incline
- •Example 3.5.2: A Spring and Rough Incline
- •3.5.1: Heat and Conservation of Energy
- •3.6: Power
- •Example 3.6.1: Rocket Power
- •3.7: Equilibrium
- •3.7.1: Energy Diagrams: Turning Points and Forbidden Regions
- •Homework for Week 3
- •Summary
- •4.1: Systems of Particles
- •Example 4.1.1: Center of Mass of a Few Discrete Particles
- •4.1.2: Coarse Graining: Continuous Mass Distributions
- •Example 4.1.2: Center of Mass of a Continuous Rod
- •Example 4.1.3: Center of mass of a circular wedge
- •4.2: Momentum
- •4.2.1: The Law of Conservation of Momentum
- •4.3: Impulse
- •Example 4.3.1: Average Force Driving a Golf Ball
- •Example 4.3.2: Force, Impulse and Momentum for Windshield and Bug
- •4.3.1: The Impulse Approximation
- •4.3.2: Impulse, Fluids, and Pressure
- •4.4: Center of Mass Reference Frame
- •4.5: Collisions
- •4.5.1: Momentum Conservation in the Impulse Approximation
- •4.5.2: Elastic Collisions
- •4.5.3: Fully Inelastic Collisions
- •4.5.4: Partially Inelastic Collisions
- •4.6: 1-D Elastic Collisions
- •4.6.1: The Relative Velocity Approach
- •4.6.2: 1D Elastic Collision in the Center of Mass Frame
- •4.7: Elastic Collisions in 2-3 Dimensions
- •4.8: Inelastic Collisions
- •Example 4.8.1: One-dimensional Fully Inelastic Collision (only)
- •Example 4.8.2: Ballistic Pendulum
- •Example 4.8.3: Partially Inelastic Collision
- •4.9: Kinetic Energy in the CM Frame
- •Homework for Week 4
- •Summary
- •5.1: Rotational Coordinates in One Dimension
- •5.2.1: The r-dependence of Torque
- •5.2.2: Summing the Moment of Inertia
- •5.3: The Moment of Inertia
- •Example 5.3.1: The Moment of Inertia of a Rod Pivoted at One End
- •5.3.1: Moment of Inertia of a General Rigid Body
- •Example 5.3.2: Moment of Inertia of a Ring
- •Example 5.3.3: Moment of Inertia of a Disk
- •5.3.2: Table of Useful Moments of Inertia
- •5.4: Torque as a Cross Product
- •Example 5.4.1: Rolling the Spool
- •5.5: Torque and the Center of Gravity
- •Example 5.5.1: The Angular Acceleration of a Hanging Rod
- •Example 5.6.1: A Disk Rolling Down an Incline
- •5.7: Rotational Work and Energy
- •5.7.1: Work Done on a Rigid Object
- •5.7.2: The Rolling Constraint and Work
- •Example 5.7.2: Unrolling Spool
- •Example 5.7.3: A Rolling Ball Loops-the-Loop
- •5.8: The Parallel Axis Theorem
- •Example 5.8.1: Moon Around Earth, Earth Around Sun
- •Example 5.8.2: Moment of Inertia of a Hoop Pivoted on One Side
- •5.9: Perpendicular Axis Theorem
- •Example 5.9.1: Moment of Inertia of Hoop for Planar Axis
- •Homework for Week 5
- •Summary
- •6.1: Vector Torque
- •6.2: Total Torque
- •6.2.1: The Law of Conservation of Angular Momentum
- •Example 6.3.1: Angular Momentum of a Point Mass Moving in a Circle
- •Example 6.3.2: Angular Momentum of a Rod Swinging in a Circle
- •Example 6.3.3: Angular Momentum of a Rotating Disk
- •Example 6.3.4: Angular Momentum of Rod Sweeping out Cone
- •6.4: Angular Momentum Conservation
- •Example 6.4.1: The Spinning Professor
- •6.4.1: Radial Forces and Angular Momentum Conservation
- •Example 6.4.2: Mass Orbits On a String
- •6.5: Collisions
- •Example 6.5.1: Fully Inelastic Collision of Ball of Putty with a Free Rod
- •Example 6.5.2: Fully Inelastic Collision of Ball of Putty with Pivoted Rod
- •6.5.1: More General Collisions
- •Example 6.6.1: Rotating Your Tires
- •6.7: Precession of a Top
- •Homework for Week 6
- •Week 7: Statics
- •Statics Summary
- •7.1: Conditions for Static Equilibrium
- •7.2: Static Equilibrium Problems
- •Example 7.2.1: Balancing a See-Saw
- •Example 7.2.2: Two Saw Horses
- •Example 7.2.3: Hanging a Tavern Sign
- •7.2.1: Equilibrium with a Vector Torque
- •Example 7.2.4: Building a Deck
- •7.3: Tipping
- •Example 7.3.1: Tipping Versus Slipping
- •Example 7.3.2: Tipping While Pushing
- •7.4: Force Couples
- •Example 7.4.1: Rolling the Cylinder Over a Step
- •Homework for Week 7
- •Week 8: Fluids
- •Fluids Summary
- •8.1: General Fluid Properties
- •8.1.1: Pressure
- •8.1.2: Density
- •8.1.3: Compressibility
- •8.1.5: Properties Summary
- •Static Fluids
- •8.1.8: Variation of Pressure in Incompressible Fluids
- •Example 8.1.1: Barometers
- •Example 8.1.2: Variation of Oceanic Pressure with Depth
- •8.1.9: Variation of Pressure in Compressible Fluids
- •Example 8.1.3: Variation of Atmospheric Pressure with Height
- •Example 8.2.1: A Hydraulic Lift
- •8.3: Fluid Displacement and Buoyancy
- •Example 8.3.1: Testing the Crown I
- •Example 8.3.2: Testing the Crown II
- •8.4: Fluid Flow
- •8.4.1: Conservation of Flow
- •Example 8.4.1: Emptying the Iced Tea
- •8.4.3: Fluid Viscosity and Resistance
- •8.4.4: A Brief Note on Turbulence
- •8.5: The Human Circulatory System
- •Example 8.5.1: Atherosclerotic Plaque Partially Occludes a Blood Vessel
- •Example 8.5.2: Aneurisms
- •Homework for Week 8
- •Week 9: Oscillations
- •Oscillation Summary
- •9.1: The Simple Harmonic Oscillator
- •9.1.1: The Archetypical Simple Harmonic Oscillator: A Mass on a Spring
- •9.1.2: The Simple Harmonic Oscillator Solution
- •9.1.3: Plotting the Solution: Relations Involving
- •9.1.4: The Energy of a Mass on a Spring
- •9.2: The Pendulum
- •9.2.1: The Physical Pendulum
- •9.3: Damped Oscillation
- •9.3.1: Properties of the Damped Oscillator
- •Example 9.3.1: Car Shock Absorbers
- •9.4: Damped, Driven Oscillation: Resonance
- •9.4.1: Harmonic Driving Forces
- •9.4.2: Solution to Damped, Driven, Simple Harmonic Oscillator
- •9.5: Elastic Properties of Materials
- •9.5.1: Simple Models for Molecular Bonds
- •9.5.2: The Force Constant
- •9.5.3: A Microscopic Picture of a Solid
- •9.5.4: Shear Forces and the Shear Modulus
- •9.5.5: Deformation and Fracture
- •9.6: Human Bone
- •Example 9.6.1: Scaling of Bones with Animal Size
- •Homework for Week 9
- •Week 10: The Wave Equation
- •Wave Summary
- •10.1: Waves
- •10.2: Waves on a String
- •10.3: Solutions to the Wave Equation
- •10.3.1: An Important Property of Waves: Superposition
- •10.3.2: Arbitrary Waveforms Propagating to the Left or Right
- •10.3.3: Harmonic Waveforms Propagating to the Left or Right
- •10.3.4: Stationary Waves
- •10.5: Energy
- •Homework for Week 10
- •Week 11: Sound
- •Sound Summary
- •11.1: Sound Waves in a Fluid
- •11.2: Sound Wave Solutions
- •11.3: Sound Wave Intensity
- •11.3.1: Sound Displacement and Intensity In Terms of Pressure
- •11.3.2: Sound Pressure and Decibels
- •11.4: Doppler Shift
- •11.4.1: Moving Source
- •11.4.2: Moving Receiver
- •11.4.3: Moving Source and Moving Receiver
- •11.5: Standing Waves in Pipes
- •11.5.1: Pipe Closed at Both Ends
- •11.5.2: Pipe Closed at One End
- •11.5.3: Pipe Open at Both Ends
- •11.6: Beats
- •11.7: Interference and Sound Waves
- •Homework for Week 11
- •Week 12: Gravity
- •Gravity Summary
- •12.1: Cosmological Models
- •12.2.1: Ellipses and Conic Sections
- •12.4: The Gravitational Field
- •12.4.1: Spheres, Shells, General Mass Distributions
- •12.5: Gravitational Potential Energy
- •12.6: Energy Diagrams and Orbits
- •12.7: Escape Velocity, Escape Energy
- •Example 12.7.1: How to Cause an Extinction Event
- •Homework for Week 12
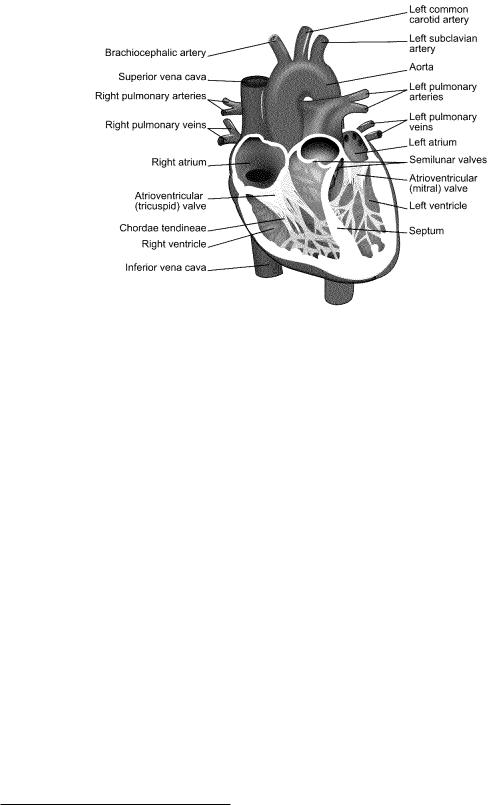
372 |
|
Week 8: Fluids |
|
|
|
|
|
|
Figure 115: A simple cut-away diagram of the human heart.
8.5: The Human Circulatory System
For once, this is a chapter that math majors, physics majors, and engineers may, if they wish, skip, although personally I think that any intellectually curious person would want to learn all sorts of things that sooner or later will impact on their own health and life. To put that rather bluntly, kids, sure now you’re all young and healthy and everything, but in thirty or forty more years (if you survive) you won’t be, and understanding the things taught in this chapter will be extremely useful to you then, if not now as you choose a lifestyle and diet that might get you through to then in reasonably good cardiovascular shape!
Here is a list of True Facts about the human cardiovascular system, in no particular order, that you should now be able to understand at least qualitatively and conceptually if not quantitatively.
•The heart, illustrated in the schematic in figure 115 above162 is the “pump” that drives blood through your blood vessels.
•The blood vessels are di erentiated into three distinct types:
–Arteries, which lead strictly away from the heart and which contain a muscular layer that elastically dilates and contracts the arteries in a synchronous way to help carry the surging waves of blood. This acts as a “shock absorber” and hence reduces the peak systolic blood pressure (see below). As people age, this muscular tissue becomes less elastic – “hardening of the arteries” – as collagen repair mechanisms degrade or plaque (see below) is deposited and systolic blood pressure often increases as a result.
159The argument won’t quite be identical – the potential di erence V there will relate to current more like the way F does to flow in our treatment, rather than P , so electrical resistance will be inversely proportional to A, not A2.
160Wikipedia: http://www.wikipedia.org/wiki/Reynolds Number.
161Wikipedia: http://www.wikipedia.org/wiki/hydraulic diameter.
162Wikipedia: http://www.wikipedia.org/wiki/Human heart. The diagram itself is borrowed from the wikipedia
creative commons, and of course you can learn a lot more of the anatomy and function of the heart and circulation by reading the wikipedia articles on the heart and following links.
Week 8: Fluids |
373 |
Arteries split up the farther one is from the heart, eventually becoming arterioles, the very small arteries that actually split o into capillaries.
–Capillaries, which are a dense network of very fine vessels (often only a single cell thick) that deliver oxygenated blood throughout all living tissue so that the oxygen can disassociate from the carrying hemoglobin molecules and di use into the surrounding cells in systemic circulation, or permit the oxygenation of blood in pulmonary circulation.
–Veins, which lead strictly back to the heart from the capillaries. Veins also have a muscle layer that expand or contract to aid in thermoregulation and regulation of blood pressure as one lies down or stands up. Veins also provide “capacitance” to the circulatory system and store the body’s “spare” blood; 60% of the body’s total blood supply is usually in the veins at any one time. Most of the veins, especially long vertical veins, are equipped with one-way venous valves every 4-9 cm that prevent backflow and pooling in the lower body during e.g. diastoli (see below).
Blood from the capillaries is collected first in venules (the return-side equivalent of arterioles) and then into veins proper.
•There are two distinct circulatory systems in humans (and in the rest of the mammals and birds):
–Systemic circulation, where oxygenated blood enters the heart via pulmonary veins from the lungs and is pumped at high pressure into systemic arteries that deliver it through the capillaries and (deoxygenated) back via systemic veins to the heart.
–Pulmonary circulation, where deoxgenated blood that has returned from the system circulation is pumped into pulmonary arteries that deliver it to the lungs, where it is oxygenated and returned to the heart by means of pulmonary veins. These two distinct circulations do not mix and together, form a closed double circulation loop.
•The heart is the pump that serves both systemic and pulmonary circulation. Blood enters into the atria and is expelled into the two circulatory system from the ventricles. Systemic circulation enters from the pulmonary veins into the left atrium, is pumped into the left ventricle through the one-way mitral valve, which then pumps the blood at high pressure into the systemic arteries via the aorta through the one-way aortic valve. It is eventually returned by the systemic veins (the superior and inferior vena cava) to the right atrium, pumped into the right ventricle through the one-way tricuspid valve, and then pumped at high pressure into the pulmonary artery for delivery to the lungs.
The human heart (as well as the hearts of birds and mammals in general) is thus fourchambered – two atria and two ventricles. The total resistance of the systemic circulation is generally larger than that of the pulmonary circulation and hence systemic arterial blood pressure must be higher than pulmonary arterial blood pressure in order to maintain the same flow. The left ventricle (primary systemic pump) is thus typically composed of thicker and stronger muscle tissue than the right ventricle. Certain reptiles also have four-chambered hearts, but their pulmonary and systemic circulations are not completely distinct and it is thought that their hearts became four-chambered by a di erent evolutionary pathway.
•Blood pressure is generally measured and reported in terms of two numbers:
–Systolic blood pressure. This is the peak/maximum arterial pressure in the wave pulse generated that drives systemic circulation. It is measured in the (brachial artery of the) arm, where it is supposed to be a reasonably accurate reflection of peak aortic pressure just outside of the heart, where, sadly, it cannot easily be directly measured without resorting to invasive methods that are, in fact, used e.g. during surgery.
–Diastolic blood pressure. This is the trough/minimum arterial pressure in the wave pulse of systemic circulation.

374 |
Week 8: Fluids |
Blood pressure has historically been measured in millimeters of mercury, in part because until fairly recently a sphygnomometer built using an integrated mercury barometer was by far the most accurate way to measure blood pressure, and it still extremely widely used in situations where high precision is required. Recall that 760 mmHg (torr) is 1 atm or 101325 Pa.
“Normal” Systolic systemic blood pressure can fairly accurately be estimated on the basis of the distance between the heart and the feet; a distance on the order of 1.5 meters leads to a pressure di erence of around 0.15 atm or 120 mmHg.
Blood is driven through the relatively high resistance of the capillaries by the di erence in arterial pressure and venous pressure. The venous system is entirely a low pressure return; its peak pressure is typically order of 0.008 bar (6 mmHg). This can be understood and predicted by the mean distance between valves in the venous system – the pressure di erence between one valve and another (say) 8 cm higher is approximately ρbg × 0.08 ≈= 0.008 bar. However, this pressure is not really static – it varies with the delayed pressure wave that causes blood to surge its way up, down, or sideways through the veins on its way back to the atria of the heart.
This di erence in pressure means one very important thing. If you puncture or sever a vein, blood runs out relatively slowly and is fairly easily staunched, as it is driven by a pressure only a small bit higher than atmospheric pressure. Think of being able to easily plug a small leak in a glass of water (where the fluid height is likely very close to 8-10 cm) by putting your finger over the hole. When blood is drawn, a vein in e.g. your arm is typically tapped, and afterwards the hole almost immediately seals well enough with a simple clot su cient to hold in the venous pressure.
If you puncture an artery, on the other hand, especially a large artery that still has close to the full systolic/diastolic pressure within it, blood spurts out of it driven by considerable pressure, the pressure one might see at the bottom of a barrel or a back yard swimming pool. Not so easy to stop it with light pressure from a finger, or seal up with a clot! It is proportionately more di cult to stop arterial bleeding and one can lose considerable blood volume in a very short time, leading to a fatal hypotension. Of course if one severs a large artery or vein (so that clotting has no chance to work) this is a very bad thing, but in general always worse for arteries than for veins, all other things being equal.
An exception of sorts is found in the jugular vein (returning from the brain). It has no valves because it is, after all, downhill from the brain back to the heart! As a consequence of this a human who is inverted (suspended by their feet, standing on their head) experiences a variety of circulatory problems in their head. Blood pools in the head, neck and brain until blood pressure there (increased by distension of the blood vessels) is enough to lift the blood back up to the heart without the help of valves, increasing venous return pressure in the brain itself by a factor of 2 to 4. This higher pressure is transmitted back throughout the brain’s vasculature, and, if sustained, can easily cause aneurisms or ruptures of blood vessels (see below)) and death from blood clots or stroke. It can also cause permanent blindness as circulation through the eyes is impaired163
Note, however, that blood is pushed through the systemic and pulmonary circulation in waves
163
”You are old, Father William,” the young man said, ”And your hair has become very white;
And yet you incessantly stand on your Do you think, at your age, it is right?”
”In my youth,” Father William replied to his son, ”I feared it might injure the brain;
But now that I’m perfectly sure I have none, Why, I do it again and again.”
Not really that good an idea...

Week 8: Fluids |
375 |
of peak pressure that actually propagate faster than the flow and that the elastic aorta acts like a balloon that is constantly refilled during the left ventricular constraction and “bu ers” the arterial pressure – in fact, it behaves a great deal like a capacitor in an RC circuit behaves if it is being driven by a series of voltage pulses! Even this model isn’t quite adequate. The systolic pulse propagates down through the (elastic) arteries (which dilate and contract to accomodate and maintain it) at a speed faster than the actual fluid velocity of the blood in the arteries. This e ectively rapidly transports a new ejection volume of blood out to the arterioles to replace the blood that made it through the capillaries in the previous heartbeat and maintain a positive pressure di erence across the capillary system even during diastole so that blood continues to move forward around the circulation loop.
Unfortunately we won’t learn the math to be able to better understand this (and be able to at least qualitatively plot the expected time variation of flow) until next semester, so in the meantime we have to be satisfied with this heuristic explanation based on a dynamical “equilibrium” picture of flow plus a bit of intuition as to how an elastic “balloon” can store pressure pulses and smooth out their delivery.
There is a systolic peak in the blood pressure delivered to the pulmonary system as well, but it is much lower than the systolic systemic pressure, because (after all) the lungs are at pretty much the same height as the heart. The pressure di erence needs only to be high enough to maintain the same average flow as that in the systemic circulation through the much lower resistance of the capillary network in the lungs. Typical healthy normal resting pulmonary systole pressures are around 15 mmHg; pressures higher than 25 mmHg are likely to be associated with pulmonary edema, the pooling of fluid in the interstitial spaces of the lungs. This is a bad thing.
The return pressure in the pulmonary veins (to the right atrium, recall) is 2-15 mmHg, roughly consistent with central (systemic) venous pressure at the left atrium but with a larger variation.
The actual rhythm of the heartbeat is as follows. Bearing in mind that it is a continuous cycle, “first” blood from the last beat, which has accumulated in the left and right atrium during the heart’s resting phase between beats, is expelled through the mitral and tricuspid valves from the left and right atrium respectively. The explusion is accomplished by the contraction of the atrial wall muscles, and the backflow of blood into the venous system is (mostly) prevented by the valves of the veins although there can be some small regurgitation. This expulsion which is nearly simultaneous with mitral barely leading tricuspid pre-compresses the blood in the ventricles and is the first “lub” one hears in the “lub-dub” of the heartbeat observed with a stethoscope.
Immediately following this a (nearly) simultanous contraction of the ventricular wall muscles causes the expulsion of the blood from the left and right ventricles through the semilunar valves into the aorta and pulmonary arteries, respectively. As noted above, the left-ventricular wall is thicker and stronger and produces a substantially higher peak systolic pressure in the aorta and systemic circulation than the right ventricle produces in the pulmonary arteries. The elastic aorta distends (reducing the peak systemic pressure as it stores the energy produced by the heartbeak) and partially sustains the higher pressure across the capillaries throughout the resting phase. This is the “dub” in the “lub-dub” of the heartbeat.
This isn’t anywhere near all of the important physics in the circulatory system, but it should be enough for you to be able to understand the basic plumbing well enough to learn more later164. Let’s do a few very important examples of how things go wrong in the circulatory system and how fluid physics helps you understand and detect them!
164...And understand why we spent time learning to solve first order di erential equations this semester so that we can understand RC circuits in detail next semester so we can think back and further understand the remarks about how the aorta acts like a capacitor this semester.