
- •Preface
- •Textbook Layout and Design
- •Preliminaries
- •See, Do, Teach
- •Other Conditions for Learning
- •Your Brain and Learning
- •The Method of Three Passes
- •Mathematics
- •Summary
- •Homework for Week 0
- •Summary
- •1.1: Introduction: A Bit of History and Philosophy
- •1.2: Dynamics
- •1.3: Coordinates
- •1.5: Forces
- •1.5.1: The Forces of Nature
- •1.5.2: Force Rules
- •Example 1.6.1: Spring and Mass in Static Force Equilibrium
- •1.7: Simple Motion in One Dimension
- •Example 1.7.1: A Mass Falling from Height H
- •Example 1.7.2: A Constant Force in One Dimension
- •1.7.1: Solving Problems with More Than One Object
- •Example 1.7.4: Braking for Bikes, or Just Breaking Bikes?
- •1.8: Motion in Two Dimensions
- •Example 1.8.1: Trajectory of a Cannonball
- •1.8.2: The Inclined Plane
- •Example 1.8.2: The Inclined Plane
- •1.9: Circular Motion
- •1.9.1: Tangential Velocity
- •1.9.2: Centripetal Acceleration
- •Example 1.9.1: Ball on a String
- •Example 1.9.2: Tether Ball/Conic Pendulum
- •1.9.3: Tangential Acceleration
- •Homework for Week 1
- •Summary
- •2.1: Friction
- •Example 2.1.1: Inclined Plane of Length L with Friction
- •Example 2.1.3: Find The Minimum No-Skid Braking Distance for a Car
- •Example 2.1.4: Car Rounding a Banked Curve with Friction
- •2.2: Drag Forces
- •2.2.1: Stokes, or Laminar Drag
- •2.2.2: Rayleigh, or Turbulent Drag
- •2.2.3: Terminal velocity
- •Example 2.2.1: Falling From a Plane and Surviving
- •2.2.4: Advanced: Solution to Equations of Motion for Turbulent Drag
- •Example 2.2.3: Dropping the Ram
- •2.3.1: Time
- •2.3.2: Space
- •2.4.1: Identifying Inertial Frames
- •Example 2.4.1: Weight in an Elevator
- •Example 2.4.2: Pendulum in a Boxcar
- •2.4.2: Advanced: General Relativity and Accelerating Frames
- •2.5: Just For Fun: Hurricanes
- •Homework for Week 2
- •Week 3: Work and Energy
- •Summary
- •3.1: Work and Kinetic Energy
- •3.1.1: Units of Work and Energy
- •3.1.2: Kinetic Energy
- •3.2: The Work-Kinetic Energy Theorem
- •3.2.1: Derivation I: Rectangle Approximation Summation
- •3.2.2: Derivation II: Calculus-y (Chain Rule) Derivation
- •Example 3.2.1: Pulling a Block
- •Example 3.2.2: Range of a Spring Gun
- •3.3: Conservative Forces: Potential Energy
- •3.3.1: Force from Potential Energy
- •3.3.2: Potential Energy Function for Near-Earth Gravity
- •3.3.3: Springs
- •3.4: Conservation of Mechanical Energy
- •3.4.1: Force, Potential Energy, and Total Mechanical Energy
- •Example 3.4.1: Falling Ball Reprise
- •Example 3.4.2: Block Sliding Down Frictionless Incline Reprise
- •Example 3.4.3: A Simple Pendulum
- •Example 3.4.4: Looping the Loop
- •3.5: Generalized Work-Mechanical Energy Theorem
- •Example 3.5.1: Block Sliding Down a Rough Incline
- •Example 3.5.2: A Spring and Rough Incline
- •3.5.1: Heat and Conservation of Energy
- •3.6: Power
- •Example 3.6.1: Rocket Power
- •3.7: Equilibrium
- •3.7.1: Energy Diagrams: Turning Points and Forbidden Regions
- •Homework for Week 3
- •Summary
- •4.1: Systems of Particles
- •Example 4.1.1: Center of Mass of a Few Discrete Particles
- •4.1.2: Coarse Graining: Continuous Mass Distributions
- •Example 4.1.2: Center of Mass of a Continuous Rod
- •Example 4.1.3: Center of mass of a circular wedge
- •4.2: Momentum
- •4.2.1: The Law of Conservation of Momentum
- •4.3: Impulse
- •Example 4.3.1: Average Force Driving a Golf Ball
- •Example 4.3.2: Force, Impulse and Momentum for Windshield and Bug
- •4.3.1: The Impulse Approximation
- •4.3.2: Impulse, Fluids, and Pressure
- •4.4: Center of Mass Reference Frame
- •4.5: Collisions
- •4.5.1: Momentum Conservation in the Impulse Approximation
- •4.5.2: Elastic Collisions
- •4.5.3: Fully Inelastic Collisions
- •4.5.4: Partially Inelastic Collisions
- •4.6: 1-D Elastic Collisions
- •4.6.1: The Relative Velocity Approach
- •4.6.2: 1D Elastic Collision in the Center of Mass Frame
- •4.7: Elastic Collisions in 2-3 Dimensions
- •4.8: Inelastic Collisions
- •Example 4.8.1: One-dimensional Fully Inelastic Collision (only)
- •Example 4.8.2: Ballistic Pendulum
- •Example 4.8.3: Partially Inelastic Collision
- •4.9: Kinetic Energy in the CM Frame
- •Homework for Week 4
- •Summary
- •5.1: Rotational Coordinates in One Dimension
- •5.2.1: The r-dependence of Torque
- •5.2.2: Summing the Moment of Inertia
- •5.3: The Moment of Inertia
- •Example 5.3.1: The Moment of Inertia of a Rod Pivoted at One End
- •5.3.1: Moment of Inertia of a General Rigid Body
- •Example 5.3.2: Moment of Inertia of a Ring
- •Example 5.3.3: Moment of Inertia of a Disk
- •5.3.2: Table of Useful Moments of Inertia
- •5.4: Torque as a Cross Product
- •Example 5.4.1: Rolling the Spool
- •5.5: Torque and the Center of Gravity
- •Example 5.5.1: The Angular Acceleration of a Hanging Rod
- •Example 5.6.1: A Disk Rolling Down an Incline
- •5.7: Rotational Work and Energy
- •5.7.1: Work Done on a Rigid Object
- •5.7.2: The Rolling Constraint and Work
- •Example 5.7.2: Unrolling Spool
- •Example 5.7.3: A Rolling Ball Loops-the-Loop
- •5.8: The Parallel Axis Theorem
- •Example 5.8.1: Moon Around Earth, Earth Around Sun
- •Example 5.8.2: Moment of Inertia of a Hoop Pivoted on One Side
- •5.9: Perpendicular Axis Theorem
- •Example 5.9.1: Moment of Inertia of Hoop for Planar Axis
- •Homework for Week 5
- •Summary
- •6.1: Vector Torque
- •6.2: Total Torque
- •6.2.1: The Law of Conservation of Angular Momentum
- •Example 6.3.1: Angular Momentum of a Point Mass Moving in a Circle
- •Example 6.3.2: Angular Momentum of a Rod Swinging in a Circle
- •Example 6.3.3: Angular Momentum of a Rotating Disk
- •Example 6.3.4: Angular Momentum of Rod Sweeping out Cone
- •6.4: Angular Momentum Conservation
- •Example 6.4.1: The Spinning Professor
- •6.4.1: Radial Forces and Angular Momentum Conservation
- •Example 6.4.2: Mass Orbits On a String
- •6.5: Collisions
- •Example 6.5.1: Fully Inelastic Collision of Ball of Putty with a Free Rod
- •Example 6.5.2: Fully Inelastic Collision of Ball of Putty with Pivoted Rod
- •6.5.1: More General Collisions
- •Example 6.6.1: Rotating Your Tires
- •6.7: Precession of a Top
- •Homework for Week 6
- •Week 7: Statics
- •Statics Summary
- •7.1: Conditions for Static Equilibrium
- •7.2: Static Equilibrium Problems
- •Example 7.2.1: Balancing a See-Saw
- •Example 7.2.2: Two Saw Horses
- •Example 7.2.3: Hanging a Tavern Sign
- •7.2.1: Equilibrium with a Vector Torque
- •Example 7.2.4: Building a Deck
- •7.3: Tipping
- •Example 7.3.1: Tipping Versus Slipping
- •Example 7.3.2: Tipping While Pushing
- •7.4: Force Couples
- •Example 7.4.1: Rolling the Cylinder Over a Step
- •Homework for Week 7
- •Week 8: Fluids
- •Fluids Summary
- •8.1: General Fluid Properties
- •8.1.1: Pressure
- •8.1.2: Density
- •8.1.3: Compressibility
- •8.1.5: Properties Summary
- •Static Fluids
- •8.1.8: Variation of Pressure in Incompressible Fluids
- •Example 8.1.1: Barometers
- •Example 8.1.2: Variation of Oceanic Pressure with Depth
- •8.1.9: Variation of Pressure in Compressible Fluids
- •Example 8.1.3: Variation of Atmospheric Pressure with Height
- •Example 8.2.1: A Hydraulic Lift
- •8.3: Fluid Displacement and Buoyancy
- •Example 8.3.1: Testing the Crown I
- •Example 8.3.2: Testing the Crown II
- •8.4: Fluid Flow
- •8.4.1: Conservation of Flow
- •Example 8.4.1: Emptying the Iced Tea
- •8.4.3: Fluid Viscosity and Resistance
- •8.4.4: A Brief Note on Turbulence
- •8.5: The Human Circulatory System
- •Example 8.5.1: Atherosclerotic Plaque Partially Occludes a Blood Vessel
- •Example 8.5.2: Aneurisms
- •Homework for Week 8
- •Week 9: Oscillations
- •Oscillation Summary
- •9.1: The Simple Harmonic Oscillator
- •9.1.1: The Archetypical Simple Harmonic Oscillator: A Mass on a Spring
- •9.1.2: The Simple Harmonic Oscillator Solution
- •9.1.3: Plotting the Solution: Relations Involving
- •9.1.4: The Energy of a Mass on a Spring
- •9.2: The Pendulum
- •9.2.1: The Physical Pendulum
- •9.3: Damped Oscillation
- •9.3.1: Properties of the Damped Oscillator
- •Example 9.3.1: Car Shock Absorbers
- •9.4: Damped, Driven Oscillation: Resonance
- •9.4.1: Harmonic Driving Forces
- •9.4.2: Solution to Damped, Driven, Simple Harmonic Oscillator
- •9.5: Elastic Properties of Materials
- •9.5.1: Simple Models for Molecular Bonds
- •9.5.2: The Force Constant
- •9.5.3: A Microscopic Picture of a Solid
- •9.5.4: Shear Forces and the Shear Modulus
- •9.5.5: Deformation and Fracture
- •9.6: Human Bone
- •Example 9.6.1: Scaling of Bones with Animal Size
- •Homework for Week 9
- •Week 10: The Wave Equation
- •Wave Summary
- •10.1: Waves
- •10.2: Waves on a String
- •10.3: Solutions to the Wave Equation
- •10.3.1: An Important Property of Waves: Superposition
- •10.3.2: Arbitrary Waveforms Propagating to the Left or Right
- •10.3.3: Harmonic Waveforms Propagating to the Left or Right
- •10.3.4: Stationary Waves
- •10.5: Energy
- •Homework for Week 10
- •Week 11: Sound
- •Sound Summary
- •11.1: Sound Waves in a Fluid
- •11.2: Sound Wave Solutions
- •11.3: Sound Wave Intensity
- •11.3.1: Sound Displacement and Intensity In Terms of Pressure
- •11.3.2: Sound Pressure and Decibels
- •11.4: Doppler Shift
- •11.4.1: Moving Source
- •11.4.2: Moving Receiver
- •11.4.3: Moving Source and Moving Receiver
- •11.5: Standing Waves in Pipes
- •11.5.1: Pipe Closed at Both Ends
- •11.5.2: Pipe Closed at One End
- •11.5.3: Pipe Open at Both Ends
- •11.6: Beats
- •11.7: Interference and Sound Waves
- •Homework for Week 11
- •Week 12: Gravity
- •Gravity Summary
- •12.1: Cosmological Models
- •12.2.1: Ellipses and Conic Sections
- •12.4: The Gravitational Field
- •12.4.1: Spheres, Shells, General Mass Distributions
- •12.5: Gravitational Potential Energy
- •12.6: Energy Diagrams and Orbits
- •12.7: Escape Velocity, Escape Energy
- •Example 12.7.1: How to Cause an Extinction Event
- •Homework for Week 12
Week 7: Statics |
321 |
position of the center of mass of the deck.
X |
|
|
− |
W |
|
− |
|
|
|
|
|
|
|
|
|
|
|||
|
|
L |
|
|
|
||||
τx |
= |
W F2 |
|
2 |
|
M g |
|
hy mg = 0 |
(665) |
X τy |
= |
hxmg + |
|
M g − LF4 = 0 |
(666) |
||||
2 |
Note that if F3 was acting, it would contribute to both of these torques and to the force above, and there would be an infinite number of possible solutions. As it is, though, solving this is pretty easy. Solve the last two equations for F2 and F4 respectively, then substitute the result into F1. Only at the end substitute numbers in and see roughly what F1 might be. Bear in mind that 1000 kg is a “metric ton” and weighs roughly 2200 pounds. So the deck and hot tub together, in this not-too-realistic problem, weigh over 6 tons!
Oops, we forgot the people in the hot tub and the barbecue grill at the far end and the furniture and the dog and the dancing. Better make the corner posts twice as strong as they need to be. Or even four times.
Once you see how this one goes, you should be ready to tackle the homework problem involving three legs, a tabletop, and a weight – same problem, really, but more complicated numbers.
7.3: Tipping
Another important application of the ideas of static equilibrium is to tipping problems. A tipping problem is one where one uses the ideas of static equilibrium to identify the particular angle or force combination that will marginally cause some object to tip over. Sometimes this is presented in the context of objects on an inclined plane, held in place by static friction, and a tipping problem can be combined with a slipping problem: determining if a block placed on an incline that is gradually raised tips first or slips first.
The idea of tipping is simple enough. An object placed on a flat surface is typically stable as long as the center of gravity is vertically inside the edges that are in contact with the surface, so that the torque created by the gravitational force around this limiting pivot is opposed by the torque exerted by the (variable) normal force.
That’s all there is to it! Look at the center of gravity, look at the corner or edge intuition tells you the object will “tip over”, done.
Example 7.3.1: Tipping Versus Slipping
In figure 97 a rectangular block of height H and width W is sitting on a rough plank that is gradually being raised at one end (so the angle it makes with the horizontal, θ, is slowly increasing).
At some angle we know that the block will start to slide. This will occur because the normal force is decreasing with the angle (and hence, so is the maximum force static friction can exert) and at the same time, the component of the weight of the object that points down the incline is increasing. Eventually the latter will exceed the former and the block will slide.
However, at some angle the block will also tip over. We know that this will happen because the normal force can only prevent the block from rotating clockwise (as drawn) around the pivot consisting of the lower left corner of the block. Unless the block has a magnetic lower surface, or a lower surface covered with velcro or glue, the plank cannot attract the lower surface of the block and prevent it from rotating counterclockwise.
As long as the net torque due to gravity (about this lower left pivot point) is into the page, the plank itself can exert a countertorque out of the page su cient to keep the block from rotating down
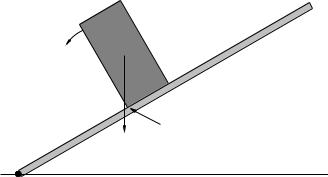
322 |
Week 7: Statics |
W
H
pivot
θ
Figure 97: A rectangular block either tips first or slips (slides down the incline) first as the incline is gradually increased. Which one happens first? The figure is show with the block just past the tipping angle.
through the plank. If the torque due to gravity is out of the page – as it is in the figure 97 above, when the center of gravity moves over and to the vertical left of the pivot corner – the normal force exerted by the plank cannot oppose the counterclockwise torque of gravity and the block will fall over.
The tipping point, or tipping angle is thus the angle where the center of gravity is directly over the pivot that the object will “tip” around as it falls over.
A very common sort of problem here is to determine whether some given block or shape will tip first or slip first. This is easy to find. First let’s find the slipping angle θs. Let “down” mean “down the incline”. Then:
X Fdown |
= |
mg sin(θ) − Fs = 0 |
(667) |
|||
X F |
= |
N − mg cos(θ) = 0 |
(668) |
|||
From the latter, as usual: |
|
|
|
|
|
|
N = mg cos(θ) |
|
|
|
(669) |
||
and Fs ≤ Fsmax = µsN . |
|
|
|
|
|
|
When |
|
|
|
|
|
|
mg sin(θ |
) = F max = µ cos(θ |
) |
(670) |
|||
s |
|
s |
s |
s |
|
|
the force of gravity down the incline precisely balances the force of static friction. We can solve for the angle where this occurs:
θs = tan−1(µs) |
(671) |
Now let’s determine the angle where it tips over. As noted, this is where the torque to to gravity around the pivot that the object will tip over changes sign from in the page (as drawn, stable) to out of the page (unstable, tipping over). This happens when the center of mass passes directly over the pivot.
From inspection of the figure (which is drawn very close to the tipping point) it should be clear that the tipping angle θt is given by:
θt = tan−1 |
µ H ¶ |
(672) |
|
|
|
W |
|
So, which one wins? The smaller of the two, θs or θt, of course – that’s the one that happens first as the plank is raised. Indeed, since both are inverse tangents, the smaller of:
µs, W/H |
(673) |

Week 7: Statics |
323 |
determines whether the system slips first or tips first, no need to actually evaluate any tangents or inverse tangents!
Example 7.3.2: Tipping While Pushing
W
F
s H h
Figure 98: A uniform rectangular block with dimensions W by H (which has its center of mass at W/2, H/2) is pushed at a height h by a force F . The block sits on a horizontal smooth table with coe cient of static friction µs.
A uniform block of mass M being pushed by a horizontal outside force (say, a finger) a height h above the flat, smooth surface it is resting on (say, a table) as portrayed in figure 98. If it is pushed down low (small h) the block slides. If pushed up high (large h) it tips. Find a condition for the height h at which it tips and slips at the same time.
The solution here is much the same as the solution to the previous problem. We independently determine the condition for slipping, as that is rather easy, and then using the maximum force that can be applied without it quite slipping, find the height h at which the block barely starts to tip over.
“Tipping over” in this case means that all of the normal force will be concentrated right at the pivot corner (the one the block will rotate around as it tips over) because the rest of the bottom surface is barely starting to leave the ground. All of the force of static friction is similarly concentrated at this one point. This is convenient to us, since neither one will therefore contribute to the torque around this point!
Conceptually, then, we seek the point h where, pushing with the maximum non-slipping force, the torque due to gravity alone is exactly equal to the torque exerted by the force F . This seems simple enough.
To find the force we need only to examine the usual force balance equations:
|
|
F − Fs |
= |
0 |
|
(674) |
|
|
N − M g |
= |
0 |
|
(675) |
and hence N = M g (as usual) and: |
|
|
|
|
|
|
F |
max |
= F max = µ N = µ |
M g |
(676) |
||
|
s |
s |
s |
|
|
(also as usual). Hopefully by now you had this completely solved in your head before I even wrote it all down neatly and were saying to yourself “Fmax, yeah, sure, that’s µsM g, let’s get on with it...”
So we shall. Consider the torque around the bottom right hand corner of the block (which is clearly and intuitively the “tipping pivot” around which the block will rotate as it falls over when the torque due to F is large enough to overcome the torque of gravity). Let us choose the positive direction for torque to be out of the page. It should then be quite obvious that when the block is barely tipping over, so that we can ignore any torque due to N and Fs:
W M g |
− hcritFmax = |
W M g |
− hcritµsM g = 0 |
(677) |
|
|
|||
2 |
2 |