
- •Preface
- •Textbook Layout and Design
- •Preliminaries
- •See, Do, Teach
- •Other Conditions for Learning
- •Your Brain and Learning
- •The Method of Three Passes
- •Mathematics
- •Summary
- •Homework for Week 0
- •Summary
- •1.1: Introduction: A Bit of History and Philosophy
- •1.2: Dynamics
- •1.3: Coordinates
- •1.5: Forces
- •1.5.1: The Forces of Nature
- •1.5.2: Force Rules
- •Example 1.6.1: Spring and Mass in Static Force Equilibrium
- •1.7: Simple Motion in One Dimension
- •Example 1.7.1: A Mass Falling from Height H
- •Example 1.7.2: A Constant Force in One Dimension
- •1.7.1: Solving Problems with More Than One Object
- •Example 1.7.4: Braking for Bikes, or Just Breaking Bikes?
- •1.8: Motion in Two Dimensions
- •Example 1.8.1: Trajectory of a Cannonball
- •1.8.2: The Inclined Plane
- •Example 1.8.2: The Inclined Plane
- •1.9: Circular Motion
- •1.9.1: Tangential Velocity
- •1.9.2: Centripetal Acceleration
- •Example 1.9.1: Ball on a String
- •Example 1.9.2: Tether Ball/Conic Pendulum
- •1.9.3: Tangential Acceleration
- •Homework for Week 1
- •Summary
- •2.1: Friction
- •Example 2.1.1: Inclined Plane of Length L with Friction
- •Example 2.1.3: Find The Minimum No-Skid Braking Distance for a Car
- •Example 2.1.4: Car Rounding a Banked Curve with Friction
- •2.2: Drag Forces
- •2.2.1: Stokes, or Laminar Drag
- •2.2.2: Rayleigh, or Turbulent Drag
- •2.2.3: Terminal velocity
- •Example 2.2.1: Falling From a Plane and Surviving
- •2.2.4: Advanced: Solution to Equations of Motion for Turbulent Drag
- •Example 2.2.3: Dropping the Ram
- •2.3.1: Time
- •2.3.2: Space
- •2.4.1: Identifying Inertial Frames
- •Example 2.4.1: Weight in an Elevator
- •Example 2.4.2: Pendulum in a Boxcar
- •2.4.2: Advanced: General Relativity and Accelerating Frames
- •2.5: Just For Fun: Hurricanes
- •Homework for Week 2
- •Week 3: Work and Energy
- •Summary
- •3.1: Work and Kinetic Energy
- •3.1.1: Units of Work and Energy
- •3.1.2: Kinetic Energy
- •3.2: The Work-Kinetic Energy Theorem
- •3.2.1: Derivation I: Rectangle Approximation Summation
- •3.2.2: Derivation II: Calculus-y (Chain Rule) Derivation
- •Example 3.2.1: Pulling a Block
- •Example 3.2.2: Range of a Spring Gun
- •3.3: Conservative Forces: Potential Energy
- •3.3.1: Force from Potential Energy
- •3.3.2: Potential Energy Function for Near-Earth Gravity
- •3.3.3: Springs
- •3.4: Conservation of Mechanical Energy
- •3.4.1: Force, Potential Energy, and Total Mechanical Energy
- •Example 3.4.1: Falling Ball Reprise
- •Example 3.4.2: Block Sliding Down Frictionless Incline Reprise
- •Example 3.4.3: A Simple Pendulum
- •Example 3.4.4: Looping the Loop
- •3.5: Generalized Work-Mechanical Energy Theorem
- •Example 3.5.1: Block Sliding Down a Rough Incline
- •Example 3.5.2: A Spring and Rough Incline
- •3.5.1: Heat and Conservation of Energy
- •3.6: Power
- •Example 3.6.1: Rocket Power
- •3.7: Equilibrium
- •3.7.1: Energy Diagrams: Turning Points and Forbidden Regions
- •Homework for Week 3
- •Summary
- •4.1: Systems of Particles
- •Example 4.1.1: Center of Mass of a Few Discrete Particles
- •4.1.2: Coarse Graining: Continuous Mass Distributions
- •Example 4.1.2: Center of Mass of a Continuous Rod
- •Example 4.1.3: Center of mass of a circular wedge
- •4.2: Momentum
- •4.2.1: The Law of Conservation of Momentum
- •4.3: Impulse
- •Example 4.3.1: Average Force Driving a Golf Ball
- •Example 4.3.2: Force, Impulse and Momentum for Windshield and Bug
- •4.3.1: The Impulse Approximation
- •4.3.2: Impulse, Fluids, and Pressure
- •4.4: Center of Mass Reference Frame
- •4.5: Collisions
- •4.5.1: Momentum Conservation in the Impulse Approximation
- •4.5.2: Elastic Collisions
- •4.5.3: Fully Inelastic Collisions
- •4.5.4: Partially Inelastic Collisions
- •4.6: 1-D Elastic Collisions
- •4.6.1: The Relative Velocity Approach
- •4.6.2: 1D Elastic Collision in the Center of Mass Frame
- •4.7: Elastic Collisions in 2-3 Dimensions
- •4.8: Inelastic Collisions
- •Example 4.8.1: One-dimensional Fully Inelastic Collision (only)
- •Example 4.8.2: Ballistic Pendulum
- •Example 4.8.3: Partially Inelastic Collision
- •4.9: Kinetic Energy in the CM Frame
- •Homework for Week 4
- •Summary
- •5.1: Rotational Coordinates in One Dimension
- •5.2.1: The r-dependence of Torque
- •5.2.2: Summing the Moment of Inertia
- •5.3: The Moment of Inertia
- •Example 5.3.1: The Moment of Inertia of a Rod Pivoted at One End
- •5.3.1: Moment of Inertia of a General Rigid Body
- •Example 5.3.2: Moment of Inertia of a Ring
- •Example 5.3.3: Moment of Inertia of a Disk
- •5.3.2: Table of Useful Moments of Inertia
- •5.4: Torque as a Cross Product
- •Example 5.4.1: Rolling the Spool
- •5.5: Torque and the Center of Gravity
- •Example 5.5.1: The Angular Acceleration of a Hanging Rod
- •Example 5.6.1: A Disk Rolling Down an Incline
- •5.7: Rotational Work and Energy
- •5.7.1: Work Done on a Rigid Object
- •5.7.2: The Rolling Constraint and Work
- •Example 5.7.2: Unrolling Spool
- •Example 5.7.3: A Rolling Ball Loops-the-Loop
- •5.8: The Parallel Axis Theorem
- •Example 5.8.1: Moon Around Earth, Earth Around Sun
- •Example 5.8.2: Moment of Inertia of a Hoop Pivoted on One Side
- •5.9: Perpendicular Axis Theorem
- •Example 5.9.1: Moment of Inertia of Hoop for Planar Axis
- •Homework for Week 5
- •Summary
- •6.1: Vector Torque
- •6.2: Total Torque
- •6.2.1: The Law of Conservation of Angular Momentum
- •Example 6.3.1: Angular Momentum of a Point Mass Moving in a Circle
- •Example 6.3.2: Angular Momentum of a Rod Swinging in a Circle
- •Example 6.3.3: Angular Momentum of a Rotating Disk
- •Example 6.3.4: Angular Momentum of Rod Sweeping out Cone
- •6.4: Angular Momentum Conservation
- •Example 6.4.1: The Spinning Professor
- •6.4.1: Radial Forces and Angular Momentum Conservation
- •Example 6.4.2: Mass Orbits On a String
- •6.5: Collisions
- •Example 6.5.1: Fully Inelastic Collision of Ball of Putty with a Free Rod
- •Example 6.5.2: Fully Inelastic Collision of Ball of Putty with Pivoted Rod
- •6.5.1: More General Collisions
- •Example 6.6.1: Rotating Your Tires
- •6.7: Precession of a Top
- •Homework for Week 6
- •Week 7: Statics
- •Statics Summary
- •7.1: Conditions for Static Equilibrium
- •7.2: Static Equilibrium Problems
- •Example 7.2.1: Balancing a See-Saw
- •Example 7.2.2: Two Saw Horses
- •Example 7.2.3: Hanging a Tavern Sign
- •7.2.1: Equilibrium with a Vector Torque
- •Example 7.2.4: Building a Deck
- •7.3: Tipping
- •Example 7.3.1: Tipping Versus Slipping
- •Example 7.3.2: Tipping While Pushing
- •7.4: Force Couples
- •Example 7.4.1: Rolling the Cylinder Over a Step
- •Homework for Week 7
- •Week 8: Fluids
- •Fluids Summary
- •8.1: General Fluid Properties
- •8.1.1: Pressure
- •8.1.2: Density
- •8.1.3: Compressibility
- •8.1.5: Properties Summary
- •Static Fluids
- •8.1.8: Variation of Pressure in Incompressible Fluids
- •Example 8.1.1: Barometers
- •Example 8.1.2: Variation of Oceanic Pressure with Depth
- •8.1.9: Variation of Pressure in Compressible Fluids
- •Example 8.1.3: Variation of Atmospheric Pressure with Height
- •Example 8.2.1: A Hydraulic Lift
- •8.3: Fluid Displacement and Buoyancy
- •Example 8.3.1: Testing the Crown I
- •Example 8.3.2: Testing the Crown II
- •8.4: Fluid Flow
- •8.4.1: Conservation of Flow
- •Example 8.4.1: Emptying the Iced Tea
- •8.4.3: Fluid Viscosity and Resistance
- •8.4.4: A Brief Note on Turbulence
- •8.5: The Human Circulatory System
- •Example 8.5.1: Atherosclerotic Plaque Partially Occludes a Blood Vessel
- •Example 8.5.2: Aneurisms
- •Homework for Week 8
- •Week 9: Oscillations
- •Oscillation Summary
- •9.1: The Simple Harmonic Oscillator
- •9.1.1: The Archetypical Simple Harmonic Oscillator: A Mass on a Spring
- •9.1.2: The Simple Harmonic Oscillator Solution
- •9.1.3: Plotting the Solution: Relations Involving
- •9.1.4: The Energy of a Mass on a Spring
- •9.2: The Pendulum
- •9.2.1: The Physical Pendulum
- •9.3: Damped Oscillation
- •9.3.1: Properties of the Damped Oscillator
- •Example 9.3.1: Car Shock Absorbers
- •9.4: Damped, Driven Oscillation: Resonance
- •9.4.1: Harmonic Driving Forces
- •9.4.2: Solution to Damped, Driven, Simple Harmonic Oscillator
- •9.5: Elastic Properties of Materials
- •9.5.1: Simple Models for Molecular Bonds
- •9.5.2: The Force Constant
- •9.5.3: A Microscopic Picture of a Solid
- •9.5.4: Shear Forces and the Shear Modulus
- •9.5.5: Deformation and Fracture
- •9.6: Human Bone
- •Example 9.6.1: Scaling of Bones with Animal Size
- •Homework for Week 9
- •Week 10: The Wave Equation
- •Wave Summary
- •10.1: Waves
- •10.2: Waves on a String
- •10.3: Solutions to the Wave Equation
- •10.3.1: An Important Property of Waves: Superposition
- •10.3.2: Arbitrary Waveforms Propagating to the Left or Right
- •10.3.3: Harmonic Waveforms Propagating to the Left or Right
- •10.3.4: Stationary Waves
- •10.5: Energy
- •Homework for Week 10
- •Week 11: Sound
- •Sound Summary
- •11.1: Sound Waves in a Fluid
- •11.2: Sound Wave Solutions
- •11.3: Sound Wave Intensity
- •11.3.1: Sound Displacement and Intensity In Terms of Pressure
- •11.3.2: Sound Pressure and Decibels
- •11.4: Doppler Shift
- •11.4.1: Moving Source
- •11.4.2: Moving Receiver
- •11.4.3: Moving Source and Moving Receiver
- •11.5: Standing Waves in Pipes
- •11.5.1: Pipe Closed at Both Ends
- •11.5.2: Pipe Closed at One End
- •11.5.3: Pipe Open at Both Ends
- •11.6: Beats
- •11.7: Interference and Sound Waves
- •Homework for Week 11
- •Week 12: Gravity
- •Gravity Summary
- •12.1: Cosmological Models
- •12.2.1: Ellipses and Conic Sections
- •12.4: The Gravitational Field
- •12.4.1: Spheres, Shells, General Mass Distributions
- •12.5: Gravitational Potential Energy
- •12.6: Energy Diagrams and Orbits
- •12.7: Escape Velocity, Escape Energy
- •Example 12.7.1: How to Cause an Extinction Event
- •Homework for Week 12
196 |
Week 4: Systems of Particles, Momentum and Collisions |
step (for physics majors or future radiologists) in understanding more advanced scattering theory but it provides a lot of direct insight into everyday experience and things like car safety and why a straight on shot in pool often stops one ball cold while the other continues on with the original ball’s velocity.
In order to be able to use momentum conservation in a collision, however, no external force can act on the colliding objects during the collision. This is almost never going to precisely be the case, so we will have to idealize by assuming that a “collision” (as opposed to a more general and leisurely force interaction) involves forces that are zero right up to where the collision starts, spike up to very large values (generally much larger than the sum of the other forces acting on the system at the time) and then drop quickly back to zero, being non-zero only in a very short time interval t.
In this idealization, collisions will (by assumption) take place so fast that any other external forces cannot significantly alter the momentum of the participants during the time t. This is called the impulse approximation. With the impulse approximation, we can neglect all other external forces (if any are present) and use momentum conservation as a key principle while analyzing or solving collisions. All collision problems solved in this course should be solved using the impulse approximation. Let’s see just what “impulse” is, and how it can be used to help solve collision problems and understand things like the forces exerted on an object by a fluid that is in contact with it.
4.3: Impulse
Let us imagine a typical collision: one pool ball approaches and strikes another, causing both balls to recoil from the collision in some (probably di erent) directions and at di erent speeds. Before they collide, they are widely separated and exert no force on one another. As the surfaces of the two (hard) balls come into contact, they “suddenly” exert relatively large, relatively violent, equal and opposite forces on each other over a relatively short time, and then the force between the objects once again drops to zero as they either bounce apart or stick together and move with a common velocity. “Relatively” here in all cases means compared to all other forces acting on the system during the collision in the event that those forces are not actually zero.
For example, when skidding cars collide, the collision occurs so fast that even though kinetic friction is acting, it makes an ignorable change in the momentum of the cars during the collision compared to the total change of momentum of each car due to the collision force. When pool balls collide we can similarly ignore the drag force of the air or frictional force exerted by the table’s felt lining for the tiny time they are in actual contact. When a bullet embeds itself in a block, it does so so rapidly that we can ignore the friction of the table on which the block sits. Idealizing and ignoring e.g. friction, gravity, drag forces in situations such as this is known as the impulse approximation, and it greatly simplifies the treatment of collisions.
~
Note that we will frequently not know the detailed functional form of the collision force, F coll(t) nor the precise amount of time t in any of these cases. The “crumpling” of cars as they collide is a very complicated process and exerts a completely unique force any time such a collision occurs – no two car collisions are exactly alike. Pool balls probably do exert a much more reproducible and understandable force on one another, one that we we could model if we were advanced physicists or engineers working for a company that made billiard tables and balls and our livelihoods depended on it but we’re not and it doesn’t. Bullets embedding themselves in blocks again do so with a force that is di erent every time that we can never precisely measure, predict, or replicate.
In all cases, although the details of the interaction force are unknown (or even unknowable in any meaningful way), we can obtain or estimate or measure some approximate things about the forces in any given collision situation. In particular we can put reasonable limits on t and make ‘before’ and ‘after’ measurements that permit us to compute the average force exerted over this time.
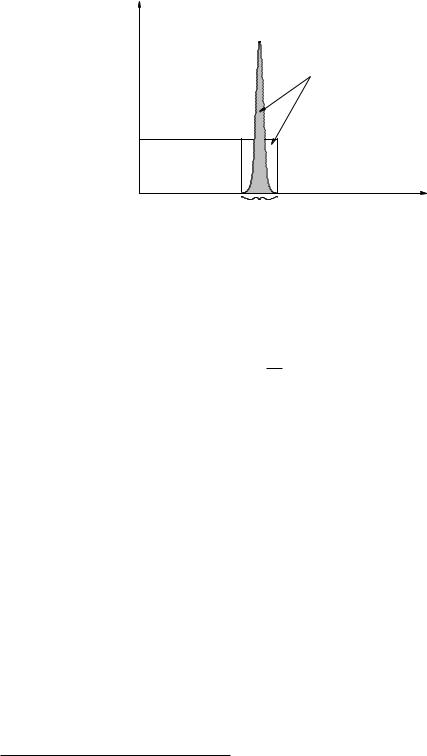
Week 4: Systems of Particles, Momentum and Collisions |
197 |
|
Let us begin, then, by defining the average force over the (short) time |
t of any given collision, |
|
~ |
~ |
|
assuming that we did know F = F 21(t), the force one object (say m1) exerts on the other object
(m2). The magnitude of such a force (one perhaps appropriate to the collision of pool balls) is sketched below in figure 51 where for simplicity we assume that the force acts only along the line of contact and is hence e ectively one dimensional in this direction97.
F(t) |
|
|
Area is |
p = |
I (impulse) |
Favg |
|
|
t |
|
t |
|
|
Figure 51: A “typical” collision force that might be exerted by the cue ball on the eight ball in a game of pool, approximately along the line connecting the two ball centers. In this case we would expect a fairly symmetric force as the two balls briefly deform at the point of contact. The time of contact t has been measured to be on the order of a tenth of a millisecond for colliding pool balls.
The time average of this force is computed the same way the time average of any other timedependent quantity might be:
~ 1 Z t ~
F avg = F (t) dt
t 0
We can evaluate the integral using Newton’s Second Law expressed in terms of momentum:
~ |
dp~ |
F (t) = |
|
dt |
|
so that (multiplying out by dt and integrating): |
|
Z t
~
p~2f − p~2i = p~2 = F (t) dt
0
(388)
(389)
(390)
This is the total vector momentum change of the second object during the collision and is also
~
the area underneath the F (t) curve (for each component of a general force – in the figure above we assume that the force only points along one direction over the entire collision and the change in the momentum component in this direction is then the area under the drawn curve). Note that the momentum change of the first ball is equal and opposite. From Newton’s Third Law,
~ |
~ |
~ |
|
|
|
|
|
|
|
|
F |
12(t) = −F |
21(t) = F and: |
|
p~1 = −Z0 |
|
|
|
|
|
|
|
|
p~1f − p~1i = |
t F~ (t) dt = − p~2 |
|
|
(391) |
||||
|
|
~ |
|
|
|
|
|
98 |
imparted by the force |
|
|
The integral of a force F over an interval of time is called the impulse |
|
||||||||
|
|
I~ = Zt1 |
F~ (t) dt = Zt1 |
dt dt = Zp1 |
dp~ = p~2 − p~1 = |
p~ |
(392) |
|||
|
|
t2 |
|
t2 |
dp~ |
p2 |
|
|
|
|
97This is, as anyone who plays pool knows from experience, an excellent assumption and is in fact how one most generally “aims” the targeted ball (neglecting all of the various fancy tricks that can alter this assumption and the outcome).
98Wikipedia: http://www.wikipedia.org/wiki/Impulse (physics).

198 |
Week 4: Systems of Particles, Momentum and Collisions |
This proves that the (vector) impulse is equal to the (vector) change in momentum over the same time interval, a result known as the impulse-momentum theorem. From our point of view, the impulse is just the momentum transferred between two objects in a collision in such a way that the total momentum of the two is unchanged.
Returning to the average force, we see that the average force in terms of the impulse is just:
~ |
~ |
|
p |
|
p~f − p~i |
|
I |
|
|
|
|||
F avg = |
|
= |
|
= |
|
(393) |
t |
t |
t |
If you refer again to figure 51 you can see that the area under Favg is equal the area under the actual force curve. This makes the average force relatively simple to compute or estimate any time you know the change in momentum produced by a collision and have a way of measuring or assigning an e ective or average time t per collision.
Example 4.3.1: Average Force Driving a Golf Ball
A golf ball leaves a 1 wood at a speed of (say) 70 meters/second (this is a reasonable number – the world record as of this writing is 90 meters/second). It has a mass of 45 grams. The time of contact has been measured to be t = 0.0005 seconds (very similar to a collision between pool balls). What is the magnitude of the average force that acts on the golf ball during this “collision”?
This one is easy:
Favg = |
I |
= |
mvf − m(0) |
= |
|
3.15 |
= 6300 Newtons |
(394) |
|
t |
t |
0.0005 |
|||||||
|
|
|
|
|
Since I personally have a mass conveniently (if embarrassingly) near 100 kg and therefore weigh 1000 Newtons, the golf club exerts an average force of 6.3 times my weight, call it 3/4 of a ton. The peak force, assuming an impact shape for F (t) not unlike that pictured above is as much as two English tons (call it 17400 Newtons).
Note Well! Impulse is related to a whole spectrum of conceptual mistakes students often make! Here’s an example that many students would get wrong before they take mechanics and that no student should ever get wrong after they take mechanics! But many do. Try not to be one of them...
Example 4.3.2: Force, Impulse and Momentum for Windshield and Bug
There’s a song by Mary Chapin Carpenter called “The Bug” with the refrain:
Sometimes you’re the windshield,
Sometimes you’re the bug...
In a collision between (say) the windshield of a large, heavily laden pickup truck and a teensy little yellowjacket wasp, answer the following qualitative/conceptual questions:
a)Which exerts a larger (magnitude) force on the other during the collision?
b)Which changes the magnitude of its momentum more during the collision?
c)Which changes the magnitude of its velocity more during the collision?
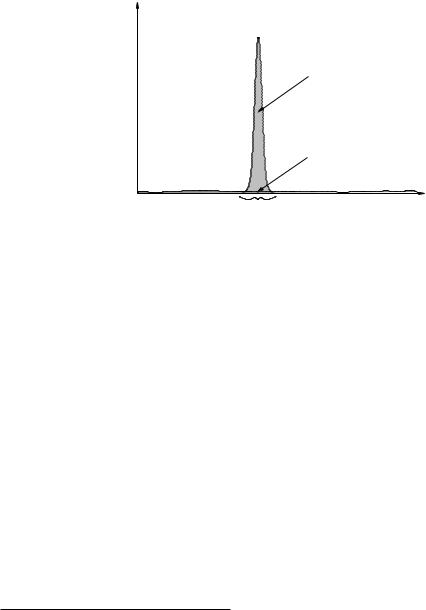
Week 4: Systems of Particles, Momentum and Collisions |
199 |
Think about it for a moment, answer all three in your mind. Now, compare it to the correct answers below99. If you did not get all three perfectly correct then go over this whole chapter until you do – you may want to discuss this with your favorite instructor as well.
4.3.1: The Impulse Approximation
When we analyze actual collisions in the real world, it will almost never be the case that there are no external forces acting on the two colliding objects during the collision process. If we hit a baseball with a bat, if two cars collide, if we slide two air-cushioned disks along a tabletop so that they bounce o of each other, gravity, friction, drag forces are often present. Yet we will, in this textbook, uniformly assume that these forces are irrelevant during the collision.
F(t) |
|
|
pc (collision) |
|
pb(background forces) |
t |
t |
|
Figure 52: Impulse forces for a collision where typical external forces such as gravity or friction or drag forces are also present.
Let’s see why (and when!) we can get away with this. Figure 52 shows a typical collision force (as before) for a collision, but this time shows some external force acting on the mass at the same time. This force might be varying friction and drag forces as a car brakes to try to avoid a collision on a bumpy road, for example. Those forces may be large, but in general they are very small compared to the peak, or average, collision force between two cars. To put it in perpective, in the example above we estimated that the average force between a golf ball and a golf club is over 6000 newtons during the collision – around six times my (substantial) weight. In contrast, the golf ball itself weighs much less than a newton, and the drag force and friction force between the golf ball and the tee are a tiny fraction of that.
If anything, the background forces in this figure are highly exaggerated for a typical collision, compared to the scale of the actual collision force!
The change in momentum resulting from the background force is the area underneath its curve, just as the change in momentum resulting from the collision force alone is the area under the collision force curve.
Over macroscopic time – over seconds, for example – gravity and drag forces and friction can make a significant contribution to the change in momentum of an object. A braking car slows down. A golf ball soars through the air in a gravitational trajectory modified by drag forces. But during
99Put here so you can’t see them while you are thinking so easily. The force exerted by the truck on the wasp is exactly the same as the force exerted by the wasp on the truck (Newton’s Third Law!). The magnitude of the momentum (or impulse) transferred from the wasp to the truck is exactly the same as the magnitude of the momentum transferred from the truck to the wasp. However, the velocity of the truck does not measurably change (for the probable impulse transferred from any normal non-Mothra-scale wasp) while the wasp (as we will see below) bounces o going roughly twice the speed of the truck...