
Книги по МРТ КТ на английском языке / Functional Neuroimaging in Child Psychiatry Ernst 1 ed 2000
.pdf94 |
D. C. Rojas, P. D. Teale and M. L. Reite |
|
|
anatomical magnetic resonance imaging, and functional magnetic resonance imaging. J. Clin. Neurophysiol., 12, 406±31.
Gharib, S., Sutherling, W. W., Nakasato, N. et al. (1995). MEG and ECoG localization accuracy test. Electroencephalogr. Clin. Neurophysiol., 94, 109±14.
Hamalainen, M. S. and Sarvas, J. (1987). Feasibility of the homogeneous head model in the interpretation of neuromagnetic Welds.
Phys. Med. Biol., 32, 91±7.
Hamalainen, M. S. and Sarvas, J. (1989). Realistic conductivity geometry model of the human head for interpretation of neuromagnetic data. IEEE Trans. Biomed. Eng., 36, 165±71.
Hamalainen, M., Hari, R., Ilmoniemi, R. J., Knuutila, J. Lounasmaa, O. V. (1993). Magnetoencephalography ± theory, instrumentation, and applications to noninvasive studies of the working human brain. Rev. Mod. Phys., 65, 413±98.
Hari, R., Joutsiniemi, S. L. and Sarvas, J. (1988). Spatial resolution of neuromagnetic records: theoretical calculations in a spherical model. Electroencephalogr. Clin. Neurophysiol., 71, 64±72.
Haueisen, J., Ramon, C., Eiselt, M., Brauer, H. and Nowak, H. (1997). InXuence of tissue resistivities on neuromagnetic Welds and electric potentials studied with a Wnite element model of the head. IEEE Trans. Biomed. Eng., 44, 727±35.
Kamada, K., Moller, M., Saguer, M. et al. (1998). Localization analysis of neuronal activities in benign Rolandic epilepsy using magnetoencephalography. J. Neurolog. Sci., 154, 164±72.
Krasuski, J., Horwitz, B. and Rumsey, J. M. (1996). A survey of functional and anatomical neuroimaging techniques. In Neuroimaging: A Window to the Neurological Foundations of Learning and Behavior in Children, eds. G. R. Lyon and J. M. Rumsey, pp. 25±52. Baltimore, MD: Paul H. Brookes.
Kullmann, W. H. (1993). Separation of sources of neuromagnetic examinations. Physiol. Meas., 14, A27±34.
Lainhart, J. E., Piven, J., Wzorek, M. et al. (1997). Macrocephaly in children and adults with autism. J. Am. Acad. Child Adolesc. Psychiatry, 36, 282±90.
Lewine, J. D. and Orrison, W. W. Jr (1995a). Clinical electroencephalography and event-related potentials. In Functional Brain Imaging, eds. W. W. Orrison, J. D. Lewine, J. A. Sanders and M. F. Hartshorne, pp. 327±68. St Louis, MO: Mosby.
Lewine, J. D. and Orrison, W. W. Jr (1995b). Magnetic source imaging: basic principles and applications in neuroradiology.
Acad. Radiol., 2, 436±40.
Lewine, J. D. and Orrison, W. W. Jr (1995c). Magnetoencephalography and magnetic source imaging. In Functional Brain Imaging, eds. W. W. Orrison, J. D. Lewine, J. A. Sanders and M. F. Hartshorne, pp. 369±418. St Louis, MO: Mosby.
Lewine, J. D. and Orrison, W. W. Jr (1995d). Spike and slow wave localization by magnetoencephalography. Neuroimaging Clin. N. Am., 5, 575±96.
Lopes da Silva, F. H., Wieringa, H. J. and Peters, M. J. (1991). Source localization of EEG versus MEG: empirical comparison using visually evoked responses and theoretical considerations. Brain Topogr., 4, 133±42.
Lü, Z.-L., Williamson, S. J. and Kaufman, L. (1992). Behavioral life-
time of human auditory sensory memory predicted by physiological measures. Science, 258, 1668±70.
Menninghaus, E., Lutkenhoner, B. and Gonzalez, S. L. (1994). Localization of a dipolar source in a skull phantom: realistic versus spherical model. IEEE Trans. Biomed. Eng., 41, 986±9.
Minami, T., Gondo, K., Yanai, S., Yamamoto, T., Tasaki, K. and Ueda, K. (1995). Rolandic discharges and somatosensory evoked potentials in benign childhood partial epilepsy: magnetoencephalographical study. Psychiatr. Clin. Neurosci., 48, S227±8.
Minami, T., Gondo, K., Yamamoto, T., Yanai, S., Tasaki, K. and Ueda, K. (1996). Magnetoencephalographic analysis of rolandic discharges in benign childhood epilepsy. Ann. Neurol. 39, 326±34.
Mosher, J. C., Lewis, P. S. and Leahy, R. M. (1992). Multiple dipole modeling and localization from spatio-temporal MEG data.
IEEE Trans. Biomed. Eng., 39, 541±57.
Mosher, J. C., Spencer, M. E., Leahy, R. M. and Lewis, P. S. (1993). Error bounds for EEG and MEG dipole source localization.
Electroencephalogr. Clin. Neurophysiol., 86, 303±21.
Murro, A. M., Smith, J. R., King, D. W. and Park, Y. D. (1995). Precision of dipole localization in a spherical volume conductor: a comparison of referential EEG, magnetoencephalography and scalp current density methods. Brain Topgr., 8, 119±25.
Nakasato, N., Levesque, M. F., Barth, D. S., Baumgartner, C., Rogers, R. L. and Sutherling, W. W. (1994). Comparisons of MEG, EEG, and ECoG source localization in neocortical partial epilepsy in humans. Electroencephalogr. Clin. Neurophysiol., 91, 171±18.
Nobre, A. C., Allison, T. and McCarthy, G. (1994). Word recognition in the human inferior temporal lobe. Nature, 372, 260±3.
Okada, Y., Lauritzen, M. and Nicholson, C. (1987). MEG source models and physiology. Phys. Med. Biol., 32, 43±51.
Okada, Y. C., Wu, J. and Kyuhou, S. (1997). Genesis of MEG signals in a mammalian CNS structure. Electroencephalogr. Clin. Neurophysiol., 103, 474±85.
Paetau, R. (1994). Sounds trigger spikes in the Landau±KleVner syndrome. J. Clin. Neurophysiol., 11, 231±41.
Paetau, R., Kajola, M., Korkman, M., Hamalainen, M., Granstrom, M. L. and Hari, R. (1991). Landau±KleVner syndrome: epileptic activity in the auditory cortex. Neuroreport, 2, 201±14.
Paetau, R., Hamalainen, M., Hari, R. et al. (1994). Magnetoencephalographic evaluation of children and adolescents with intractable epilepsy. Epilepsia, 35, 275±84.
Paetau, R., Ahonen, A., Salonen, O. and Sams, M. (1995). Auditory evoked magnetic Welds to tones and pseudowords in healthy children and adults. J. Clin. Neurophysiol., 12, 177±85.
Reeve, A., Rose, D. F. and Weinberger, D. R. (1989). Magnetoencaphalography. Applications in psychiatry. Arch. Gen. Psychiatry, 46, 573±6.
Reite, M., Teale, P., Zimmerman, J., Davis, K., Whalen, J. and Edrich, J. (1988). Source origin of a 50-msec latency auditory evoked
Weld component in young schizophrenic men. Biol. Psychiatry,
24, 495±506.
Reite, M., Teale, P., Goldstein, L., Whalen, J. and Linnville, S. (1989). Late auditory magnetic sources may diVer in the left hemisphere of schizophrenic patients. A preliminary report. Arch. Gen. Psychiatry, 46, 565±72.

Magnetoencephalography 95
Reite, M., Sheeder, J., Teale, P. et al. (1997). Magnetic source imaging evidence of sex diVerences in cerebral lateralization in schizophrenia. Arch. Gen. Psychiatry, 54, 433±40.
Rezai, A. R., Hund, M., Kronberg, E. et al. (1995). Introduction of magnetoencaphalography to stereotactic techniques.
Stereotactic Funct. Neurosurg., 65, 37±41.
Rojas, D. C., Teale, P., Sheeder, J., Simon, J. and Reite, M. (1997). SexspeciWc expression of Heschl's gyrus functional and structural abnormalities in paranoid schizophrenia. [see comments] Am. J. Psychiatry, 154, 1655±62.
Rojas, D. C., Walker, J. R., Sheeder, J. L., Teale, P. D. and Reite, M. L. (1998). Developmental changes in refractoriness of the neuromagnetic M100 in children. Neuroreport, 9, 1261±5.
Rojas, D. C., Sheeder, J. L., Teale, P. et al. (1999). MEG measurement of auditory sensory memory persistence via the M100 in children and adults. In Advances in Biomagnetism Research: Biomag96, eds. C. Aine, Y. Okada, G. Stroink, S. Swithenby and C. Wood, New York: Springer±Verlag.
Romani, G. L. (1987). The inverse problem in MEG studies: an instrumental and analytical perspective. Phys. Med. Biol., 32, 23±31.
Salmelin, R., Service, E., Kiestla. P., Uutela, K. and Salonen, O. (1996). Impaired visual word processing in dyslexia revealed with magnetoencephalography. Anna. Neurol., 40, 157±62.
Sanders, J. A. and Orrison, W. W. (1995). Functional magnetic resonance imaging. In Functional Brain Imaging, eds. W. W. Orrison, J. D. Lewine, J. A. Sanders. and M. F. Hartshorne, pp. 239±326. St Louis, MO: Mosby.
Sarvas, J. (1987). Basic mathematical and electromagnetic concepts of the biomagnetic inverse problem. Phys. Med. Biol., 1, 11±22.
Scherg, M., Hari, R. and Hamalainen, M. (1989). FrequencyspeciWc sources of the auditory N19±P30±P50 response detected by a multiple source analysis of evoked magnetic Welds and potentials. In Advances in Biogmagnetism, eds. M. Hoke, G. Stroink and M. Kotani, pp. 97±100. New York: Plenum.
Turner, R. and Jezzard, P. (1994). Magnetic resonance studies of brain functional activation using echo-planar imaging. In
Functional Neuroimaging Technical Foundations, eds. R. W. Thatcher, M. Hallet, T. ZeYro, E. R. John and M. Huerta, pp. 69±78. San Diego, CA: Academic Press.
van den Broek, S. P., Reinders, F., Donderwinkel, M. and Peters, M. J. (1998). Volume conduction eVects in EEG and MEG.
Electroencaphalogr. Clin. Neurophysiol., 106, 522±34.
Vanni, S., Uusitalo, M. A., Kiesila, P. and Hari, R. (1997). Visual motion activates V5 in dyslexics. Neuroreport, 8, 1939±42.
Virtanen, J., Ahveninen, J., Ilmoniemi, R. J., Näätänen, R. and Pekkonen, E. (1998). Replicability of MEG and EEG measures of the auditory N1/N1m-response. Electroencaphalogr. Clin. Neurophysiol., 108, 291±8.
Wakai, R. T., Leuthold, A. C. and Martin, C. B. (1996). Fetal auditory evoked responses detected by magnetoencephalography. Am. J. Obst. Gynaecol., 174, 1484±6.
Williamson, S. J. and Kaufman, L. (1990). Theory of neuroelectric and neuromagnetic Welds. In Auditory Evoked Magnetic Fields and Electric Potentials, Vol. 6, eds. F. Grandori, M. Hoke and G. L. Romani, pp. 1±39. New York: Karger.
Witelson, S. F. and Pallie, W. (1973). Left hemisphere specialization for language in the newborn: neuroanatomical evidence of asymmetry. Brain, 96, 641±6.
Wood, M. L. (1992). Fourier imaging. In Magnetic Resonance Imaging, eds. D. D. Stark and W. G. Bradley, St Louis, MO: Mosby Year Book.
Woodhouse, W., Bailey, A., Rutter, M., Bolton, P., Baird, G. and Le Couteur, A. (1996). Head circumference in autism and other pervasive developmental disorders. J. Child Psychol. Psychiatry, 37, 665±71.
Zhang, Y., Tavrin, Y., Muck, M. et al. (1993a). High temperature RF SQUIDS for biomedical applications. Phsyiol. Meas., 14, 113±19.
Zhang, Y., Tavrin, Y., Muck, M. et al. (1993b). Magnetoencephalography using high temperature rf SQUIDs. Brain Topogr., 5, 379±82.
Zupanc, M. L. (1997). Neuroimaging in the evaluation of children and adolescents with intractable epilepsy: II. Neuroimaging and pediatric epilepsy surgery. Pediatr. Neurol., 17, 111±21.
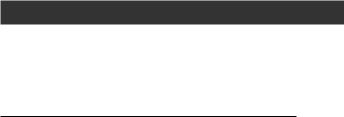
Part 2
Ethical foundations
Critical to the application of these exciting technologies within child psychiatry are ethical considerations. Ethics is central to all research, but special issues arise when dealing with subjects who are particularly vulnerable by virtue of their age and dependence, as well as by virtue of impairments resulting from psychiatric disorders. In this section, Arnold and colleagues outline the guiding ethical principles in research with children and address the challenges speciWc to functional neuroimaging studies of both normal control children and those with psychiatric disorders. SpeciWc risks imposed by functional neuroimaging and informed consent/ assent issues are examined, and methods for minimizing such risks are described.
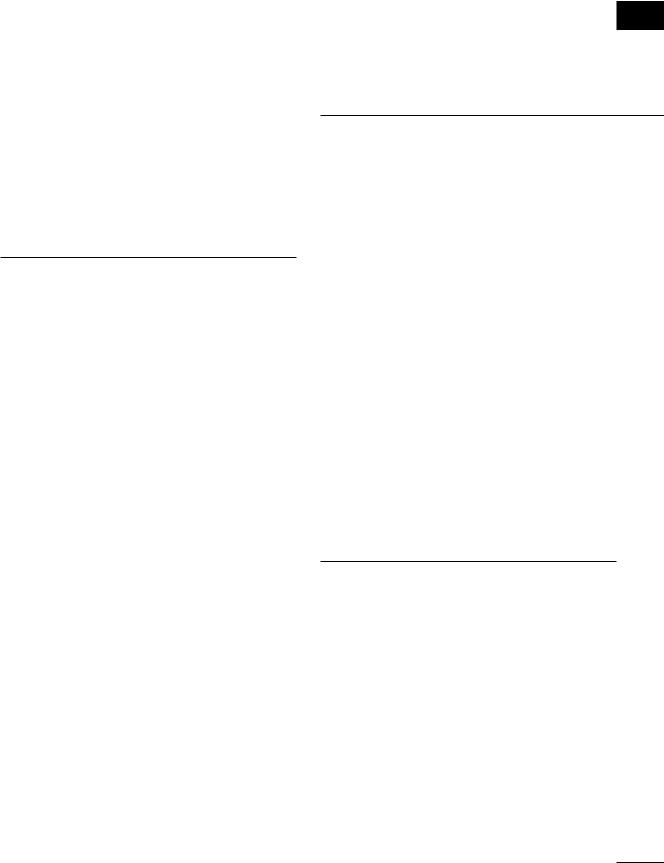
6
Ethical issues in neuroimaging research with children
L. Eugene Arnold, Alan J. Zametkin, Lauren Caravella and Nicole Korbly
Introduction
The ªDecade of the Brainº has witnessed a burgeoning of neuroimaging in psychiatric research with children and adolescents. A 1994 survey of the research use of various biological procedures reported in Wve major journals over a 5-year period revealed 18 reports based on brain imaging, constituting 15% of the reports of biological procedures (Arnold et al., 1995, 1996). Nine of these reports (8%) employed procedures utilizing ionizing radiation. The use of such procedures has increased and has become more frequent in children and adolescents. As with much other research technology, neuroimaging was initially used only in adults and is being extended to minors. Its application to children and adolescents raises additional ethical issues involving both risks and beneWts, which will be reviewed here.
Children have often been ªresearch orphansº whose exclusion from research has limited their ability to beneWt from it. With the recent adoption by the National Institutes of Health (NIH) of a policy encouraging the inclusion of children in research, their role in neuroimaging research is now entering a new era. Similar to the NIH policy on the inclusion of women and minorities in research, the new policy for the inclusion of children requires explicit justiWcation whenever minors are to be excluded from research. Both policies are designed to extend the beneWts of research to groups that have been historically neglected by the scientiWc community.
Throughout this review, the three principles for the protection of human subjects of scientiWc research, established in the Belmont report (1979), will be honored. They include:
1Respect for persons, which recognizes the dignity and autonomy of individuals and requires protection for people with diminished autonomy (such as young chil-
dren). This principle is honored through the use of informed consent and assent.
2 BeneWcence, which requires optimizing beneWts and minimizing risks. This principle is reXected by the careful assessment of beneWt±risk ratio and the requirement of a favorable ratio to allow research to proceed.
3Justice, which refers to the ªfairness of distributionº of the burdens and beneWts of research. This principle needs to be considered in the selection of subjects. In fact, the recent NIH initiative regarding the need to justify excluding minors in research stems in part from this principle. The controversial inclusion of healthy children as control subjects in research is also to be examined in light of this principle.
Of these three principles, that of beneWcence, with the assessment of beneWt±risk ratio, poses the most complex issues.
BeneWt_ risk ratio: principle of beneWcence
Human subjects protections and children
As in any research endeavour, neuroimaging research must minimize the experimental risks to the participants. A given experimental procedure may carry a risk of diVerent magnitude in children than in adults, because of children's additional vulnerabilities. These vulnerabilities include sensitivity to novelty, few previous experiences, incompletely developed ability to understand the altruistic nature of research, and such physiologic vulnerabilities as smaller size, faster metabolism, and potentially altered sensitivity to some medication side eVects.
Because of children's special vulnerabilities, society has established special legal and ethical protections for them, including protection from research risk. This generally
99

100L. E. Arnold, A. J. Zametkin, L. Caravella and N. Korbly
adds to the safeguards aVorded by the natural protection of parents. In fact, society sometimes implies that parents' judgment may not be adequately protective of their children. To insure protection, society may act as a superparent by limiting the rights of parents to make decisions for their children. For example, in some settings the legal concept of in loco parentis is invoked to justify societal authorities making decisions that ordinarily would fall to a parent. The US federal research safeguards for children (45 Code of Federal Regulation (CFR), Subtitle A, Part 46, Subpart D, 10-1-91 Edition) indicate that parents' or guardians' permission for research on their children may not be solicited or accepted for research with substantially greater than minimal risk unless there is direct beneWt to the child with a beneWt±risk ratio at least as good as available alternatives. Therefore, the concept of ªminimal riskº is crucial for research in children.
DeWnition of minimal risk
There is much debate over the deWnition of minimal risk, which Kopelman (1989) characterizes as pivotal. ªRiskº invokes a measure of both the probability and the magnitude of potential harm. The threshold deWning ªminimal riskº can vary greatly among institutional review boards (IRBs) because of its ambiguous meaning. A deWnition is suggested by 45 Code of Federal Regulation (CFR), Subtitle A, Part 46, Subpart A, 46.102(g) 10-1-91 Edition: ª`Minimal risk' means that the risks of harm anticipated in the proposed research are not greater, considering probability and magnitude, than those ordinarily encountered in daily life or during the performance of routine physical or psychological examinations or tests.º This deWnition seems to accept as minimal the risks that are comparable to the risks of everyday activities that parents and society routinely approve for children, such as bike riding, riding in a car, and even seasonal activities such as swimming or sled riding. However, authorities are not clear about this nor about the deWnitions of ªordinaryº or ªroutineº.
Unfortunately, there are many ways to interpret the oYcial deWnition of minimal risk above. Kopelman (1989) points out that the phrase ªordinarily encountered in daily lifeº can be interpreted as (i) all the risks ordinary people encounter, (ii) the risks all people ordinarily encounter, (iii) or the minimal risks all ordinary people ordinarily encounter. We should note that research is not considered an ordinary daily risk, but rather an additional risk.
Another problem is that what is routine for one child may not be routine risk or discomfort for another child. A question to be resolved is whether a given procedure represents minimal risk for both patients and health controls. How do
the daily risks experienced by a patient with a disorder compare with the daily risks of a control subject? On the one hand, it might be argued that a patient who has daily exposure to electrolyte imbalance or malnutrition from an eating disorder, or a patient who clinically requires daily administration of risky medications, may encounter more risks in daily life than control subjects. On the other hand, it could be argued that the state of vulnerability from these risks makes a patient less able to tolerate additional research risks.
Nicholson (1986) has proposed a list of examples of ordinary daily risks to which research risks can be compared to determine minimal risk. Such a list may be helpful as long as it is used only for guidance and not interpreted rigidly.
Risk and aversiveness
The process of deWning minimal risk requires clariWcation of the relationship between aversiveness and risk. Both are negative outcomes and one may cause the other. A procedure can be risky without being aversive or uncomfortable, or can be aversive without being risky (at least physically). However, extreme aversiveness constitutes a psychologic risk (e.g., adjustment disorder or post-traumatic stress disorder), especially if subjects are not provided careful support. For some anxiety-prone children, the experience of being enclosed in a magnetic resonance imaging (MRI) scanner could conceivably be psychologically traumatic, especially without adequate preparation.
The obvious need for individual evaluation of aversiondriven psychologic risks is partly met by the requirement for child assent in addition to parent permission. By deWnition and federal statute, minors are required toªassentº to participate in research and only adults (i.e., parents) can consent to their own or their children's participation. If the procedure is extremely aversive or perceived as aversive, assent will presumably not be forthcoming. The same procedure may be less aversive as part of a research protocol than when conducted under routine clinical necessity. Research investigators typically invest time and eVort in psychologic preparation to maintain participant cooperation.The pressure of clinical emergencies and stringencies of cost containment often prohibit the same degree of preparation for clinical patients. Several authors (Jay et al., 1982; Castellanos et al., 1994; Rosenberg et al., 1997) have documented that there is an opportunity to minimize the psychologic risk of aversiveness by preliminary rehearsal or role playing.
Risks of neuroimaging
A literature search yielded few systematic studies of the physical or psychologic risks of biological research proce-
Ethical issues in neuroimaging research with children |
101 |
|
|
dures in general, and none on neuroimaging in children. The relevant clinical literature about risks and side eVects is somewhat richer. The risks involved in neuroimaging research in children include those associated with radiation exposure, electromagnetic Welds, sedation or anesthesia, and psychologic stress (e.g., claustrophobic reaction, exposure to a hospital setting).
Techniques for studying the brain in vivo have evolved rapidly during the past decades, beginning with X-ray computed tomography (CT) in the late 1960s to early 1970s. Each type of imaging carries its own special risks. The history of CT scan use illustrates the potential of research to reduce risks. Before systematic neuroimaging research, many clinicians routinely obtained CT scans as part of their assessment of developmental disorders such as autism. A beneWt±risk assessment, promoted by research studies, suggested this practice to be inappropriate and led to its discontinuation.
Radiation exposure
The health risks of radiation exposure at the doses used in positron emission tomography (PET) and single photon emission computed tomography (SPECT) studies have been extensively reviewed by Ernst et al. (1998). The major concerns lie with the question of whether the levels of radiation exposure involved in these techniques increase a subject's risk for developing cancer.
The review by Ernst and co-workers (1998) of the largest studies of radiation risks, measured as the probability of excess rates of cancer among populations exposed to lowdose radiation (deWned as less than 20 or 10rems), concluded that an excess risk could not be detected in association with low-level radiation exposure. The highest research radiation dose used in imaging studies of healthy children 12 years of age and older has been 0.06rem to the whole body. The rem is a unit of dose equivalent that accounts for the cellular and subcellular diVerences in the energy deposition pattern of various ray (1 rem5 0.01Sv (sievert, the SI unit)). This dose is approximately one-tenth of the dose limit recommended by the US Food and Drug Administration (FDA) for adult volunteers in research.
Because children may be more vulnerable to the eVects of radiation than are adults, the FDA restricts the use of radioactive drugs in research involving minors to a cumulative dose per year of 0.5rem (0.3 rem in single dose) to whole body, active blood-forming organs, lens of the eye, and gonads, and 1.5rem (0.5 rem in single dose) for other organs, which is one tenth of the limit for research use in adults. The International Commission on Radiological Protection (IRCP) sets general public involuntary risk guidelines at 1mSv per year (approximately 0.1rem),
which is slightly above most background levels from soil radioactivity and cosmic radiation at sea level. The IRCP sets occupational limits or whole body exposure at 5rem per year in adults. In addition, a 3rem limit for exposure to any individual organ or tissue has been established by 21 Code of Federal Regulations Ch. 1 Part 361.1. In children, the limit is set at one tenth that for adults.
The radiation eVect that confers biological risk is that of unrepaired genetic mutation. While such unrepaired mutations increase risk, it is important to note that genetic mutations are common events that are corrected physiologically by powerful cellular repair mechanisms. An average of 240000 genetic mutations occur spontaneously daily in the human body. Radiation exposure of 1rem adds about 100 more genetic mutations to this number (Billen, 1990). Although debated, there is some evidence that previous low-level radiation can have a protective eVect from subsequent high-dose radiation exposure by stimulating chromosomal repair mechanisms (for review, see Ernst et al., 1998).
Studies that expose human subjects to ionizing radiation must be reviewed by the local Radiation Safety Committee (RSC) or Radioactive Drug Review Committee (RDRC) and the IRB throughout the research. RDRCs or RSCs are composed of members with expertise in radiation biology who are competent to assess the risk of exposure. IRBs often lack this degree of expertise and must be educated both by members of the RSC and by the review of materials addressing these risks. A process of careful collaboration and consultation among researchers, RDRC, and IRB members is essential for a logical, unfragmented risk assessment, with each party contributing their speciWc expertise and taking the appropriate responsibility. The expertise of RSC and RDRC members should be sought during the conceptualization and design of the protocol, and members of the RSC and RDRC should attend IRB meetings to consult on the use of ionizing radiation. The lay perception of risk from radiation often greatly exceeds the risk measured in controlled research studies of large samples, and this bias is often harboured by members of the IRB despite otherwise excellent scientiWc credentials.
Electromagnetic ®eld exposure
Functional MRI (fMRI), magnetic resonance spectroscopy (MRS), and magnetoencephalography (MEG) all use conventional MRI scanners. Although MRI does not expose subjects to ionizing radiation risk, the potential risk of exposure to a high magnetic Weld ($1.5T) cannot be measured accurately. Some of the inherent risks in MRI studies at any magnet strength include the rare complication of scanning a patient who has had metal clips or pacemakers

102L. E. Arnold, A. J. Zametkin, L. Caravella and N. Korbly
inserted or has ingested scraps of metal (see Chapter 3). Movement of such materials induced by the magnetic Weld can have serious consequences. To prevent such occurrences, a medical history with explicit questioning of caretakers about prior surgeries and the possibility of any metallic implants should be systematically included as part of a screening. Histories that raise concern can be followed up with X-rays or other appropriate medical examinations.
Heating of tissue is a theoretical and practical concern at high Weld strengths. However, with the magnetic Welds currently used (up to 4T), adverse eVects from heating are unlikely.
In MRI-related studies, sedation probably remains the greatest risk for those who require it. At present, many IRBs have not allowed minors to participate in studies at the high magnetic Weld strengths (3±4T) that permit the fast acquisition of whole-brain fMRI scans with adequate spatial resolution and the analysis of various components of the MRS signal (e.g., the diVerentiation in MRS of the glutamatergic from the GABAergic components of the composite Glx signal).
Sedation or anesthesia for brain imaging
Sedation of minors for imaging is a routine part of pediatric practice and is associated with very low risk (Williams et al., 1997). For patients, the use of sedation in situations of no direct or immediate beneWt requires careful justiWcation but should not be categorically rejected given the low risk. For example, after review of the complication rate associated with deep sedation (propofol) of severely impaired children, an IRB approved the use of this method in PET studies of minors with autism and with Lesch±Nyhan disease, despite the lack of direct beneWt to the patients. There was no complication in those studies (Ernst et al., 1996, 1997a). In view of the catastrophic nature of pervasive development disorders, childhood-onset schizophrenia, and similarly crippling disorders, this adjunct to imaging should not stand as a rigid impediment to brain imaging. Although anesthesiologists often argue that general anesthesia with intubation is safer than deep sedation, investigators as well as IRB members will need to be better educated to decide whether the risks of general anesthesia are, in fact, lower than those of deep sedation. In some instances, sedation may be obviated by familiarizing the subjects to the research procedures. Such approaches have been used successfully in several laboratories (for example, see Chapter 13). It may be possible to enhance the beneWt±risk ratio by adding clinically indicated tests to a research procedure, such as performing a clinical MRI in the context of a brain imaging study while the participant is sedated.
Hospital/clinic setting as a stressor
Although not technically a neuroimaging procedure, an additional ªinterventionº to be considered when weighing the risks of research is the hospital visit that is sometimes necessary. Two studies have assessed whether hospitalization of pediatric subjects was related to subsequent psychiatric morbidity. Douglas (1975) found that a single hospitalization of 1 week or less of children below an age of 5 years was not associated with later behavior disturbance or poor reading in adolescence. Similarly, Quinton and Rutter (1976) found no association between one-time hospitalization of a week or more regardless of age with emotional or conduct disturbance at age 10 years. Furthermore, visits to medical centers for children and adolescents may serve to demystify this setting, particularly if the experience is positive, thus making future visits to doctors and hospitals less stressful.
Unexpected or unwanted knowledge about individuals: con®dentiality
Much like genetic studies, another problem for neuroimaging procedures is the handling of unexpected or unwanted knowledge gained in the course of research. This is especially relevant for control subjects. It appears advisable to discuss with the child and parents their wishes about possible contingencies before the results are known. Wertz et al. (1994) suggest that the child or adolescent should be the primary decision-maker about being told things with no direct health beneWts (i.e., no treatment or prevention possible) but which might be useful to the minor in making future decisions. Others advise that the parents decide whether and how to communicate the information to their child. Consent and assent forms should be explicit regarding any baseline ancillary studies to be performed, such as HIV or pregnancy testing, and should specify who will be informed in the event of positive test results. Such a pre-test discussion must be handled skillfully and reassuringly because it may create risks by arousing unnecessary anxiety. It may also be useful to solicit general policy guidance from nonresearch sources such as self-help groups. Such groups might help to formulate general guidelines about when and what individuals in various scenarios would like to be told.
The risk of breach of conWdentiality between children and parents needs to be carefully evaluated and is particularly salient in research with adolescents. For example, most functional neuroimaging protocols require research participants to have no history of illicit substance use. How the investigators will deal with this type of information needs to be carefully delineated in the protocol, and in the consent and assent forms.
Ethical issues in neuroimaging research with children |
103 |
|
|
A risk of unwanted knowledge increasingly noted by IRBs is an economic one. Evidence of previously undiagnosed chronic disease or genetic vulnerability that may be found in the course of research could prevent the subject from getting medical insurance and possibly life insurance in the future if this information became accessible. Reportedly, some IRBs have interpreted this economic risk as more than minimal and have denied approval of some protocols on this basis even though the other risks were acceptable.
Minimizing risks
Risks can be minimized by limiting the number and type of research procedures to prevent research days from being unduly long, tiresome, or anxiety provoking. In longitudinal studies, deciding the appropriate number and frequency of serial tests requires careful balancing of the incremental value of additional knowledge against incremental risk for each repetition.
Investigators have an obligation to do no more invasive tests than necessary to answer the central research questions and to devise designs that minimize the number and invasiveness of tests. Can a blood draw replace a spinal tap, or can blood be drawn while starting an intravenous line, thus minimizing the number of needle punctures? In the absence of allergy, the application of a local anaesthetic cream (e.g., one containing lidocaine (lignocaine) such as Emla cream) can be used systematically in children prior to a needle puncture. Less-invasive tests should be sought and technology devised to yield the same information for which a more invasive test might have been needed.
Another approach to minimizing risks is to modify the methodologic paradigms. For example, duration of sedation can be shortened in PET imaging by giving the sedative agent only after the uptake of the tracer in the brain has been completed (40min for Xuorodeoxyglucose, 90min for Xuorodihydroxyphenylalanine (Xuoro DOPA)). This strategy requires using a sedative with a short and reliable onset of action (like propofol). An additional advantage of this technique is that the study results, which reXect the uptake period of the tracer, are not inXuenced by the sedative agent; in this case, minimization of risk maximizes the science.
To minimize risk during the PET scanning of minors, Ernst et al. (1997b) modiWed their procedure for adults to reduce radiation exposure in minors (below the guideline for minors of 10% of the dose limit established for adults). This was accomplished by (i) reducing the amount of tracer injected; (ii) lengthening the scan acquisition time,
thus recovering image resolution lost as a result of the lower injected dose; (iii) using measures to avoid artifacts secondary to repositioning subjects in the scanner and (iv) allowing subjects to void during the study, thus removing the tracer from the bladder, which is the organ with the highest level of exposure during Xuorodeoxyglucose PET scans.
Another strategy to minimize risk in PET studies has recently become available with the advent of highly sensitive whole-body PET scanners. Prior to the availability of these large-bore cameras, any measurement of absolute levels of brain metabolism required the insertion of a radial arterial catheter for acquisition of an arterial input curve of the tracer. The insertion of arterial catheters in healthy minors for research purposes was considered by most IRBs to be ªabove minimal riskº. Actual risks of arterial catheterization have been reviewed in a follow-up NIH study of 106 subjects with arterial catheterization for PET protocols (Jons et al., 1997). This study identiWed only minor abnormal signs (e.g., transient haematoma, local redness) that would not have attracted medical attention otherwise. With large-bore PET cameras (whole-body cameras), the arterial input data can be replaced by cardiac input data obtained noninvasively by imaging the left ventricle of the heart (see Chapter 1).
Since risk and discomfort may be inversely proportional to the skill of the technician or clinician performing the procedure, the most skilled clinician should be recruited. Arranging for presence of parents, family, or peers during the procedure may be useful to allay the child's anxiety.
Another minimization of risk, or at least discomfort, involves carefully determining the value and hazards of preparatory procedures (e.g., fasting, special diets, resting, caVeine restrictions, and medication withdrawal). Are these necessary for subject safety? Or are they merely necessary for quality of test results? If the latter, is the increment of knowledge worth the increment of subject discomfort?
Risk can be further minimized by implementation of procedures to prepare the subject and monitor consequences and after-eVects (Jay et al., 1982; Kruesi et al., 1988; Castellanos et al., 1994). For example, Amiel (1985) used videotape movies to keep children comfortable while they remained in a supine position after a spinal tap, which reduced the risk of postspinal tap headache. Children may have a lower (or higher) threshold for aversive stimuli than adults, or the after-eVects of aversive stimuli may last a longer or shorter time. Studies to establish such thresholds and perceptions of aversive procedures could be useful.
A Wnal point about preparing subjects for imaging procedures is that more preparation is not necessarily better.