
Книги по МРТ КТ на английском языке / MR Imaging in White Matter Diseases of the Brain and Spinal Cord - K Sartor Massimo Filippi Nicola De Stefano Vincent Dou
.pdf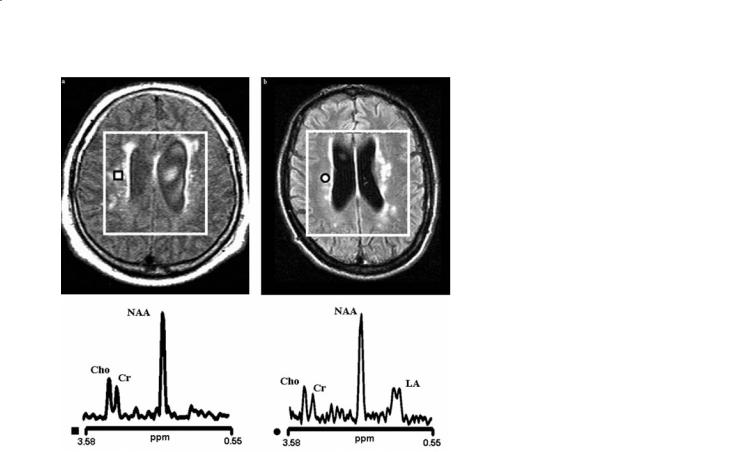
Proton MR Spectroscopy in Metabolic Disorders of the Central Nervous System
a |
|
b |
|
|
|
a |
b |
199
Fig. 13.4a,b. Conventional MRI of two elderly patients (over 70 year of age) with hypertension, loss of memory, previous cerebral transitory ischemic attacks (a, b) and their proton MR spectra coming from voxels localized to the deep periventricular white matter (square and circle, respectively). The volume of interest of the multi-voxel spectroscopic examination is shown in both images. In both patients, conventional MRI shows periventricular lesions suggestive of leukoaraiosis. In one patient (right), there is high resonance intensity of lactate (LA) in the proton spectrum. This patient was subsequently diagnosed as having MELAS (mitochondrial encephalopathy with lactic acidosis and stroke-like episodes), despite a strokelike episode never being reported in the clinical history. The other patient (left) does not show changes in lactate resonance intensity and was subsequently diagnosed as having initial cerebrovascular dementia
rons and their processes in mature brains decreases in this metabolite are interpreted as an index of axonal damage (Matthews et al. 1998; Bjartmar et al. 2002). This is most likely due to a secondary axonal dysfunction and/or loss, suggestive of the relevance of neuro-axonal damage in both demyelinating and dysmyelinating disorders. Recent studies suggest that NAA may provide a surrogate measure that can be relevant to clinical disability in patients with several white matter disorders (de Stefano et al. 2000b).
13.3
Diagnostic-Specific MRS Changes in WM Disorders
As already mentioned, most of the metabolic changes detected in white matter disorders are not diseasespecific. However, in some specific circumstances, proton MRS can offer information useful for the differential diagnosis beyond what is currently available in routine clinical use or can even provide typical brain metabolic patterns characteristic of a given disorder.
As proton MRS can provide a specific and accurate interpretation of the pathological processes un-
derlying the white matter lesions, this can be used to differentiate brain lesions appearing similar on MRI. Different pathophysiology might be seen, for example, in hypoxic-ischemic white matter lesions with a complex pathogenesis existing in rare metabolic conditions such as mitochondrial encephalopathy with lactic acidosis and stroke-like episodes (MELAS) (Dubeau et al. 2000). Patients with MELAS can show very large increases in levels of brain parenchymal Lac in the area of the acute stroke (Fig. 13.3). Also, large increases in brain parenchymal Lac can be found in the brain tissue even in patients without history of acute ischemic attack (Fig. 13.4). Thus, proton MRS findings can allow for an accurate differentiation of this maternally inherited condition from any other acute and chronic cerebrovascular disorders.
In other very rare metabolic disorders such as ethylmalonic encephalopathy (Grosso et al. 2004) and cerebrotendinous xanthomatosis (de Stefano et al. 2001), the findings of diffuse brain mitochondrial impairment have strongly contributed to the interpretation of the complex pathogenetic mechanisms of these disorders. In the first case (Grosso et al. 2004), the diffuse MRS increase in brain Lac detected in a multi-voxel MRSI study (Fig. 13.5) was underlying a primary mitochondrial disorder, as later demonstrated by biochemical and genetic studies (Coburn
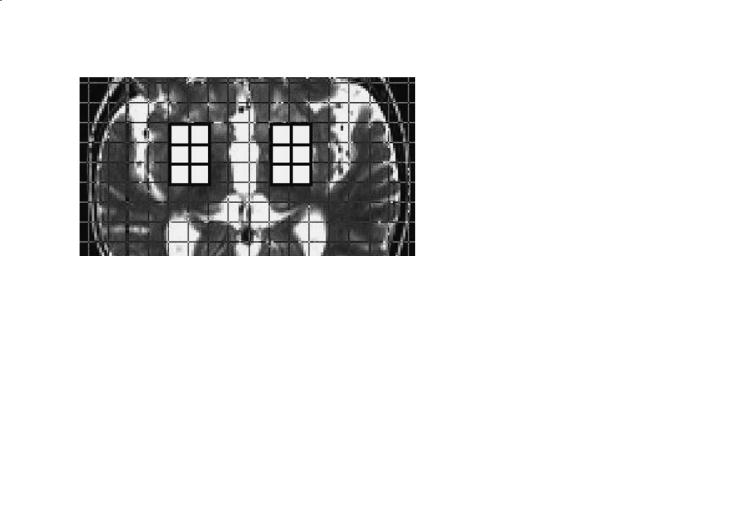
200 |
N. De Stefano and M. Mortilla |
a |
b |
Fig. 13.5a,b. Conventional T2-weighted MRI with the superimposed grid of spectroscopy voxels (a) and proton MR spectra corresponding to brain voxels located in the basal ganglia of both cerebral hemispheres (b) in a patient with ethylmalonic encephalopathy. Each spectrum shows large pathological signals at 1.33 ppm coming from the methyl doublet of lactate (Lac)
a |
b |
Fig. 13.6a,b. Single-voxel proton MR spectra relative to cerebral (a) and cerebellar (b) volume of interests of a normal control [left panels in (a) and (b)] and of a patient with cerebrotendinous xanthomatosis [right panels in (a) and (b)]. Note the cerebral and cerebellar increases in lactate in the patient’s spectra with respect to normal control
2004).In the latter condition (de Stefano et al.2001), single-voxel proton MRS data (Fig. 13.6) add to morphological and biochemical evidence of mitochondrial dysfunction (Federico et al. 1991; Dotti et al. 1995) (probably secondary to the toxic effect of high cholestanol and/or bile alcohol levels), suggesting the presence of a diffuse impairment of mitochondrial oxidative metabolism in patients with cerebrotendinous xanthomatosis.
There are also conditions in which proton MRS can provide typical brain metabolic patterns able to address the diagnosis. One example of a disease in which MRS provides a diagnostic pattern is a spongiform leukoencephalopathy known as Canavan disease. In this disorder, the deficiency of the enzyme aspartoacylase (which breaks down NAA) is responsible for abnormally high levels of NAA in the brain, which can be considered pathognomonic (Austin et al. 1991; Grodd et al. 1990). Another characteristic metabolic MRS pattern has been shown recently in other spongiform leukoencephalopathies such as megalencephalic cystic leukoencephalopathy (MCL)
(Hanefeld et al. 1993; van der Knaap et al. 1995) and childhood ataxia with diffuse CNS hypomyelination (CACH, also called vanishing white matter disease) (Tedeschi et al. 1995; van der Knaap et al. 1997). In these disorders, conventional MRI findings of extensive white matter abnormalities with sparing of central brain structures are seen together with a peculiar MRS pattern. This is characterized by the almost complete disappearance of all normally detected metabolites in the white matter, presence of small increases in Lac and sparing of gray matter that is structurally and metabolically normal. In MCL, although MRS abnormalities tend to be more pronounced with increasing age, these are generally mild and the frontal white matter is significantly less involved than other white matter regions (Fig. 13.7). In patients with CACH disease, increases in glucose resonance intensities may also be present. This MRS metabolic profile is perhaps due to little brain white matter tissue left and the great increase in extracellular spaces. In both diseases, white matter increases in Lac resonance intensities are small and inconstant

Proton MR Spectroscopy in Metabolic Disorders of the Central Nervous System |
201 |
a |
c |
b
Fig. 13.7a–c. Conventional FLAIR image and proton MR spectra corresponding to brain voxels located in the frontal white matter (a), periventricular white matter (b) and grey matter (c) in the MRI/MRSI examination performed in a patient with megalencephalic cystic leukoencephalopathy at 25 years of age. Loss of signals in all the metabolites normally detected with the long echo time multi-voxel MRSI method are evident in white matter voxels. These abnormalities are relatively mild in the frontal white matter (a) and more pronounced in the periventricular white matter (b). Moderate increases in Lac resonance intensity can be seen in both white matter regions. No abnormalities are evident in grey matter voxels (c)
suggesting that they might be due to a disturbance in Lac removal after accumulation of foamy macrophages in pathologically active areas more than to a primary impairment of the oxidative metabolism. In any case, these MRS patterns appear to be sufficiently specific to differentiate these spongiform leukoencephalopathies from other white matter disorders with similar clinical and radiological appearance.
As mentioned before, distinctive white matter changes can also be seen in a number of mitochondrial encephalopathies (Bardosi et al. 1987;
Burgeois et al. 1992; Leutner et al. 1994; Nakai et al. 1994). Significant decreases in Cho have been observed in some mitochondrial encephalopathies even in the absence of white matter abnormality on conventional MRI (Arnold and Matthews 1996; de Stefano et al. 1995c). These are likely related to be associated vacuolar myelinopathy which has been described pathologically in Kearns-Sayre syndrome and some of its variance (Matthews et al. 1993). Proton MRS studies also show decrease in NAA and increases in Lac relative resonance intensities in primitive mitochondrial encephalopathies (Matthews et al. 1993; Arnold and Matthews
1996). The latter, in particular, represents an important biochemical marker of these disorders.Lac levels are transiently increased in a number of acute pathological conditions associated with inflammatory cells
(Arnold et al. 1992; Petroff et al. 1992; Arnold and Matthews 1996), but extensive pathological increases in Lac both within and outside of MRI lesions may be indicative of widespread energy failure associated with mitochondrial dysfunction (Matthews et al. 1993; de Stefano et al. 1995c). Accordingly, classical primitive mitochondrial such as pyruvate dehydrogenase deficiency, Leigh’s and Kearns-Sayre syndromes show diffuse increases in brain parenchymal Lac (Fig. 13.8).
Other rare metabolic conditions also may provide diagnostic-specific proton MRS findings. In the phenylketonuria, patients show a specific peak due to the elevated phenylalanine and proton MRS can be used in patients with this metabolic disorder to follow the influx of phenylalanine from blood into brain tissue as well as to monitor the response of diet therapy (Kreis et al. 1995; Pietz et al. 2003). In the leukoencephalopathy associated with the disturbance of the metabolism of the polyols (van der Knaap et al. 1999), the diffuse decrease of all normally detected metabolites is associated with the increases of arabitol and sorbitol in both white and grey matter regions. In maple syrup disease, a relatively specific broad peak is detectable at 0.9 ppm.This region of the spectrum is usually attributed to lipids, but in maple syrup disease is believed to represent resonances of methyl protons from branched-chain amino-acids
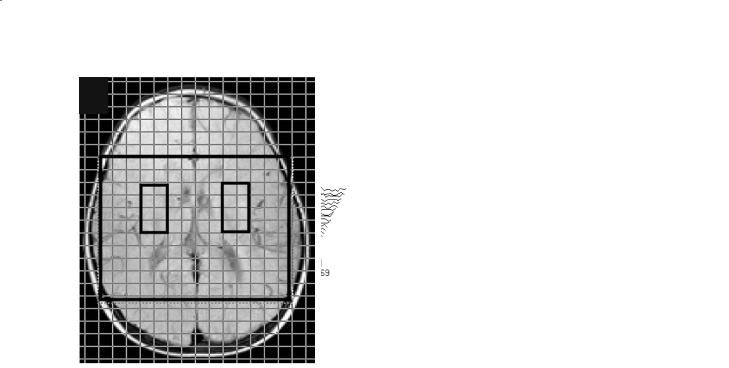
202 N. De Stefano and M. Mortilla
a b
Fig. 13.8a,b. Conventional MRI with the superimposed grid of spectroscopy voxels (a) and proton MR spectra corresponding to brain voxels located in the basal ganglia of both cerebral hemispheres (b) in a patient with pyruvate dehydrogenase deficiency. Each spectrum shows large pathological signals at 1.33 ppm coming from the methyl doublet of lactate (Lac)
and branched-chain alpha-keto acids that accumulate as a result of defective oxidative decarboxylation of leucine, isoleucine and valine (Jan et al. 2003).Also proton MRS studies on patients with Niemann-Pick type C disease have shown increased resonance intensity of the lipid region of the spectrum (Fig. 13.9), probably due, in this case, to a defective metabolism of cholesterol with ceramide accumulation (Sylvain et al. 1994; Battisti et al. 2003). In both maple syrup and Niemann-Pick type C disease, the abnormal broad peak detectable at 0.9 ppm seem to decrease with appropriate therapy (Jan et al. 2003; Sylvain et al. 1994).
Finally, specific metabolic syndromes have recently been revealed by using proton MRS. This is the case of the creatine deficiency syndromes, which include defects in the guanidinoacetate methyltransferase and in the arginine-glycine amidinotransferase (Stockler et al. 1994; Schulze 2003), and the X-linked creatine deficiency syndrome (Bizzi et al. 2002). In the first two forms of the diseases, the Cr resonance intensity is undetectable in the brain on proton MRS, but cerebral levels of Cr do increase after creatine supplementation. In the X-linked form of creatine deficiency, as the metabolic defect is due to the transport of creatine into the central nervous system, patients are unresponsive to treatment and the Cr resonance intensity levels are unchanged after creatine supplementation (Fig. 13.10). Another
condition with very specific proton MRS spectrum is the unique case of a child with minor developmental delay and absence of cerebral NAA, in whom the most prominent peak of proton MRS at 2.02 ppm was undetectable (Martin et al. 2001). Both creatine deficiency syndromes and the absence of NAA are characterized by mild or absent abnormalities on conventional MRI suggesting the unique potential of proton MRS in revealing metabolic abnormalities in MRI normal-appearing tissue.
13.4
Metabolic Changes Beyond MRI Lesions
As extensively reported in patients with multiple sclerosis (Fu et al. 1998), also in patients with both hereditary and acquired white matter disorders the detection of brain metabolic changes is not restricted to lesions (de Stefano et al. 2000b). Metabolic changes in normal-appearing white matter are usually less severe than those found inside the lesions and mostly consist in decreases in NAA and increases in Lac and Cho.
Because axons project through lesion volumes,any axonal dysfunction or loss should extend well beyond the borders of a lesion and into normal-appearing white matter, as an expression of anterograde and
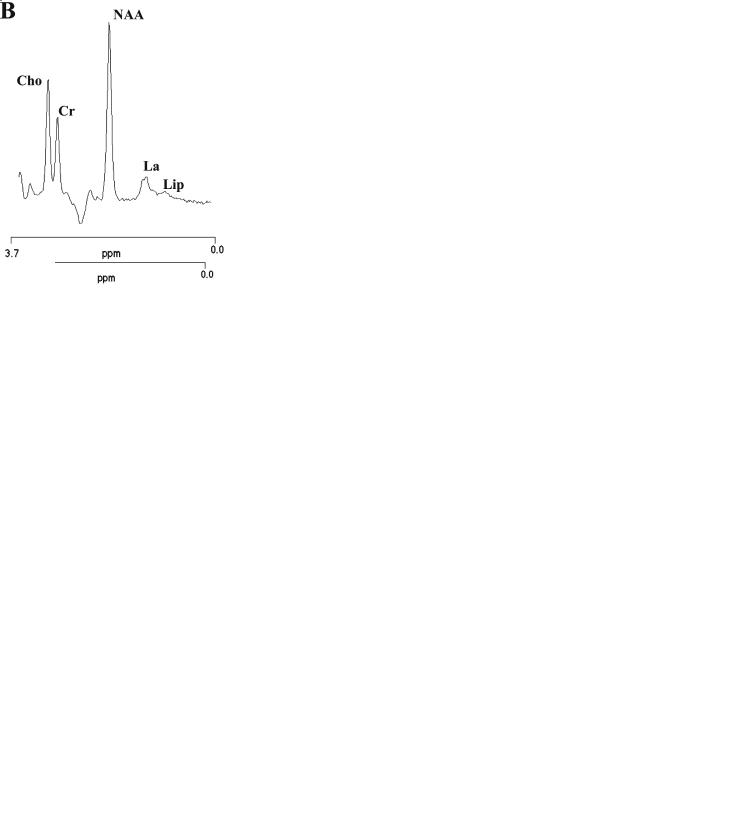
Proton MR Spectroscopy in Metabolic Disorders of the Central Nervous System |
203 |
||||
|
|
|
|
|
|
|
|
|
|
|
|
|
|
|
|
|
|
|
|
|
|
|
|
a |
b |
Fig. 13.9a,b. Single voxel proton MR spectra relative to a normal control (a) and a patient with Niemann-Pick type C disease (b). The spectra originate from homologous large volume of interest localized above the lateral ventricles and including grey and white matter of both cerebral hemispheres. Note the large decrease in N-acetyl aspartate and the increase in the lactate/lipids signals in the patient with Niemann-Pick type C disease respect to the normal control. Increases in lipids, rare in MR spectra at long echo times, are interpreted as due to ceramide accumulation
retrograde axonal degeneration. Thus, it should not be surprising that decreases in NAA can be observed beyond the borders of MS lesions as defined by T2- weighted MRI. The analysis of serial multi-voxel proton MRSI studies from patients who present with large,solitary demyelinating lesions offers special opportunities in this respect. These studies showed that decreases in NAA can be seen well outside the demyelinating lesion (de Stefano et al. 1995a) and can be transiently evident even in homologous voxels of the hemisphere contralateral to the lesion (de Stefano et al. 1999).
Increases in Lac resonance intensities in the nor- mal-appearing brain tissue represent a biochemical characteristic of mitochondrial encephalopathies. As mentioned before, Lac levels are transiently increased in a number of acute pathological conditions associated with inflammation (Arnold et al. 1992; Petroff et al. 1992), but pathological increases in Lac outside of MRI lesions may be indicative of widespread mitochondrial impairment (Matthews et al. 1993; de Stefano et al. 1995c).
Increases in Cho also could be detected in the nor- mal-appearing WM in several WM conditions. This is the case, for example, in patients with rare, inherited diseases such as adrenoleukodystrophy (Kruse et al. 1994) and adult-onset Krabbe disease (de Stefano et al. 2000a) (Fig. 13.11). In all of these cases, the detec-
a
b
c
Fig. 13.10a–c. Proton brain MR spectra of a patient with X- linked creatine deficiency. The spectra show decreases in creatine resonance intensity (a), which remains unchanged after 3 months of oral creatine monohydrate supplementation at 400 mg/kg/day (b) and after 8 months of supplementation at 800 mg/kg/day (c). (Courtesy of Alberto Bizzi)
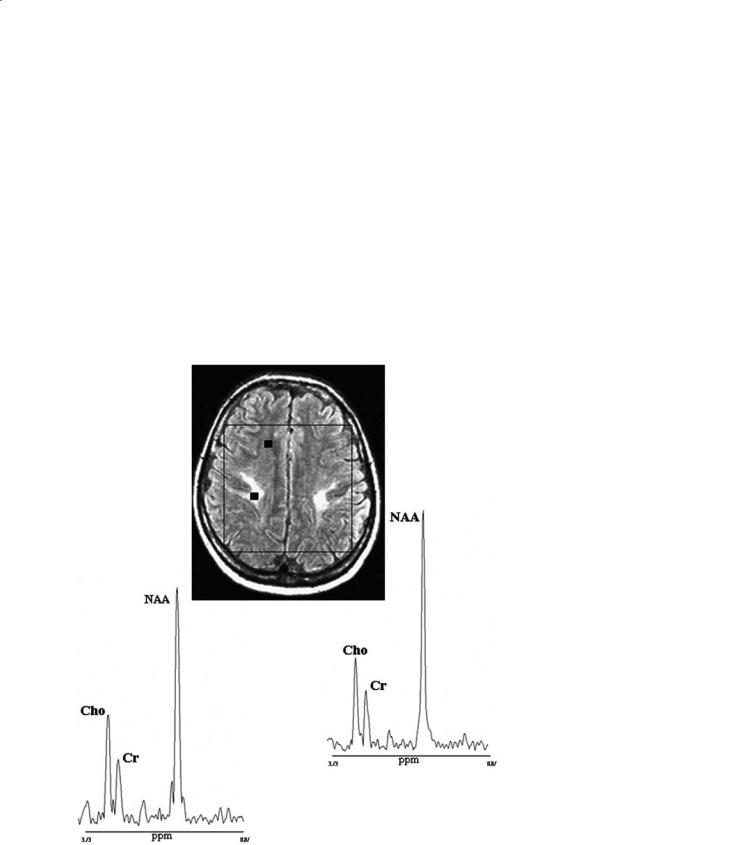
204 |
N. De Stefano and M. Mortilla |
tion of metabolic changes in tissue appearing normal on MRI should be interpreted as the first sign of white matter pathology, not yet detectable by conventional MRI.
On the other hand, brain metabolic changes sometimes can be independent of the degree of abnormalities seen on conventional MRI. This can be found, for example, in CADASIL (cerebral autosomal dominant arteriopathy with subcortical infarcts and leukoencephalopathy) patients with mild and severe neurological impairment who can show similar white matter abnormalities on conventional MRI and, respectively, mild and severe brain metabolic abnormalities on multi-voxel proton MRSI (Fig. 13.12). Similarly, a single voxel study showed that this is also valid in other diseases such as in congenital muscular dystrophy where, in a patient
with no central nervous system impairment, the MRS metabolic pattern was normal despite the diffuse white matter abnormalities on conventional MRI (Fig. 13.13). This confirms once again the lack of pathological specificity of the white matter lesions detected on conventional MRI and supports the hypothesis that brain lesions appearing similar on MRI may have a different pathophysiology and, as a consequence, different clinical relevance. In contrast, in both CADASIL and congenital muscular dystrophy as well as in other metabolic disorders cerebral levels of NAA correlated closely to patients’ clinical status (de Stefano et al. 2000b) suggesting that the widespread cerebral neuro-axonal dysfunction and/or loss represents the most relevant mechanism of functional impairment in several metabolic disorders affecting the cerebral white matter.
b
a
Fig. 13.11a,b. Proton brain MRI/MRSI examination of a patient with adult-onset Krabbe disease. Conventional MRI examination show abnormally high signal localized to the motor strips of both hemispheres. The volume of interest for spectroscopy is shown in the transverse MRI. Proton MRSI voxels were classified as inside the lesion if they were entirely filled with abnormal MRI signal (a) and as outside the lesion if there was no abnormal MRI signal within the voxel and in adjacent voxels (b). Proton MRSI spectra from voxels [squares in (a) and (b)] show abnormal increases in choline resonance intensities
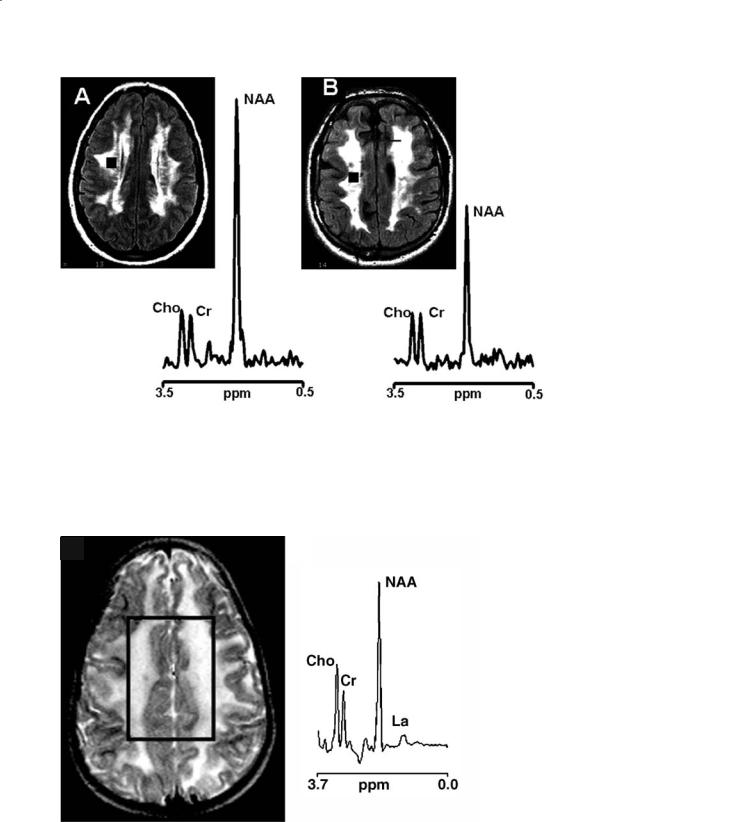
Proton MR Spectroscopy in Metabolic Disorders of the Central Nervous System |
205 |
|||
|
|
|
|
|
a |
|
b |
|
|
|
|
|
|
|
Fig. 13.12a,b. Proton brain MRI/MRSI examinations relative to two patients with cerebral autosomal dominant arteriopathy with subcortical infarcts and leukoencephalopathy (CADASIL) and mild (a) and severe (b) neurological impairment, respectively. Patients show similar white matter abnormalities on conventional MRI. In contrast, proton MRSI voxels localized in the deep white matter (squares) show metabolic changes which are independent of the degree of MRI white matter abnormalities and are very mild in the CADASIL patient with mild neurological impairment, and more pronounced in the CADASIL patient with severe clinical status
a |
b |
|
Fig. 13.13a,b. Conventional MRI in transversal orientation (a) and the single voxel proton MRS spectrum of a patient with congenital muscular dystrophy (b). The volume of interest for spectroscopy is shown in the transverse MRI. The conventional MR image shows diffuse white matter hyperintensity, but the MR spectrum is normal. The patient, despite the diffuse cerebral white matter abnormalities, did not have CNS impairment

206
13.5 Conclusions
Brain MRS provides chemical-pathological information that has the potential to improve both diagnostic classification and management of patients with metabolic disorders affecting the brain white matter. Metabolic indices provided by proton MRS and MRSI could be sensitive indicators of early neurological involvement, relevant to patients’ clinical status. A more extensive use of a combination of MR modalities including volumetric MRI, MRS and other nonconventional MR techniques might yield a more complete description of the dynamics responsible for pathological changes in this heterogeneous group of disorders and allow a more accurate evaluation of disease progression and response to therapy. A wider use of proton MRS techniques should be encouraged in clinical studies of patients with metabolic disorders.
References
Arnold DL, Matthews PM (1996) Practical aspects of clinical applications of MRS in the brain. In: Young IR, Charles HC (eds) MR spectroscopy: clinical applications and techniques. Dunitz, London, pp 139-159
Arnold DL, Matthews PM, Francis GS, O’Connor J, Antel JP (1992) Proton magnetic resonance spectroscopic imaging for metabolic characterization of demyelinating plaques. Ann Neurol 31:235-241
Austin SJ, Connelly A, Gadian DG, Benton JS, Brett EM (1991) Localized 1H NMR spectroscopy in Canavan’s disease: a report of two cases. Magn Reson Med 19:439-445
Bardosi A, Creutzfeldt W, DiMauro S, Felgenhauer K, Friede RL, Goebel HH et al (1987) Myo-, neuro-, gastrointestinal encephalopathy (MNGIE syndrome) due to partial deficiency of cytochrome-c-oxidase. A new mitochondrial multisystem disorder. Acta Neuropathol 74:248-258
Battisti C, Tarugi P, Dotti MT, De SN, Vattimo A, Chierichetti F et al (2003) Adult onset Niemann-Pick type C disease: a clinical, neuroimaging and molecular genetic study. Mov Disord 18:1405-1409
Bizzi A, Bugiani M, Salomons GS, Hunneman DH, Moroni I, Estienne M et al (2002) X-linked creatine deficiency syndrome: a novel mutation in creatine transporter gene SLC6A8. Ann Neurol 52:227-231
Bjartmar C,Battistuta J,Terada N,Dupree E,Trapp BD (2002) N- acetylaspartate is an axon-specific marker of mature white matter in vivo: a biochemical and immunohistochemical study on the rat optic nerve. Ann Neurol 51:51-58
Bruhn H, Kruse B, Korenke GC, Hanefeld F, Hanicke W, Merboldt KD et al (1992) Proton NMR spectroscopy of cerebral metabolic alterations in infantile peroxisomal disorders. J Comput Assist Tomogr 16:335-344
N. De Stefano and M. Mortilla
Burgeois M, Goutieres F, Chretien D, Rustin P, Munnich A, Aicardi J (1992) Deficiency in complex II of the respiratory chain, presenting as a leukodystrophy in two sisters with Leigh syndrome. Brain Dev 14:404-408
Coburn B (2004) A rare disorder, ethylmalonic encephalopathy, is caused by mutations in a mitochondrial protein. Clin Genet 65:460-462
Davies SE, Newcombe J, Williams SR, McDonald WI, Clark JB (1995) High resolution proton NMR spectroscopy of multiple sclerosis lesions. J Neurochem 64:742-748
De Stefano N, Matthews PM, Antel JP, Preul M, Francis G, Arnold DL (1995a) Chemical pathology of acute demyelinating lesions and its correlation with disability. Ann Neurol 38:901-909
De Stefano N, Matthews PM, Arnold DL (1995b) Reversible decreases in N-acetylaspartate after acute brain injury. Magn Reson Med 34:721-727
De Stefano N, Matthews PM, Ford B, Genge A, Karpati G, Arnold DL (1995c) Short-term dichloroacetate treatment improves indices of cerebral metabolism in patients with mitochondrial disorders. Neurology 45:1193-1198
De Stefano N, Narayanan S, Matthews PM, Francis GS, Antel JP, Arnold DL (1999) In vivo evidence for axonal dysfunction remote from focal cerebral demyelination of the type seen in multiple sclerosis. Brain 122:1933-1939
De Stefano N, Dotti MT, Mortilla M, Pappagallo E, Luzi P, Rafi MA et al (2000a) Evidence of diffuse brain pathology and unspecific genetic characterization in a patient with an atypical form of adult-onset Krabbe disease. J Neurol 247:226-228
De Stefano N, Narayanan S, Matthews PM, Mortilla M, Dotti MT, Federico A et al (2000b) Proton MR spectroscopy to assess axonal damage in multiple sclerosis and other white matter disorders. J Neurovirol 6 [Suppl 2]:121-129
De Stefano N, Dotti MT, Mortilla M, Federico A (2001) Magnetic resonance imaging and spectroscopic changes in brains of patients with cerebrotendinous xanthomatosis. Brain 124:121-131
Dotti MT, Manneschi L, Federico A (1995) Mitochondrial enzyme deficiency in cerebrotendinous xanthomatosis. J Neurol Sci 129:106-108
Dubeau F, De SN, Zifkin BG, Arnold DL, Shoubridge EA (2000) Oxidative phosphorylation defect in the brains of carriers of the tRNAleu(UUR) A3243G mutation in a MELAS pedigree. Ann Neurol 47:179-185
Federico A, Dotti MT, Volpi N (1991) Muscle mitochondrial changes in cerebrotendinous xanthomatosis (letter). Ann Neurol 30:734-735
Fu L, Matthews PM, De Stefano N,Worsley KJ, Narayanan S, Francis GS et al (1998) Imaging axonal damage of normal-appear- ing white matter in multiple sclerosis. Brain 121:103-113
Grodd W, Krageloh-Mann I, Petersen D, Trefz FK, Harzer K (1990) In vivo assessment of N-acetylaspartate in brain in spongy degeneration (Canavan’s disease) by proton spectroscopy (letter). Lancet 336:437-438
Grosso S, Balestri P, Mostardini R, Federico A, De SN (2004) Brain mitochondrial impairment in ethylmalonic encephalopathy. J Neurol 251:755-756
Hanefeld F, Holzbach U, Kruse B, Wilichowski E, Christen HJ, Frahm J (1993) Diffuse white matter disease in three children: an encephalopathy with unique features on magnetic resonance imaging and proton magnetic resonance spectroscopy. Neuropediatrics 24:244-248

Proton MR Spectroscopy in Metabolic Disorders of the Central Nervous System |
207 |
Jan W, Zimmerman RA, Wang ZJ, Berry GT, Kaplan PB, Kaye EM (2003) MR diffusion imaging and MR spectroscopy of maple syrup urine disease during acute metabolic decompensation. Neuroradiology 45:393-399
Johannik K, Van Hecke P, Francois B, Marchal G, Smet MH, Jaeken J et al (1994) Localized brain proton NMR spectroscopy in young adult phenylketonuria patients. Magn Reson Med 31:53-57
Kolodny EH (1993) Dysmyelinating and demyelinating conditions in infancy (review). Curr Opin Neurol Neurosurg 6:379-386
Koopmans RA, Li DK, Zhu G,Allen PS, Penn A, Paty DW (1993) Magnetic resonance spectroscopy of multiple sclerosis: in-vivo detection of myelin breakdown products (letter). Lancet 341:631-632
Kreis R, Pietz J, Penzien J, Herschkowitz N, Boesch C (1995) Identification and quantitation of phenylalanine in the brain of patients with phenylketonuria by means of localized in vivo 1H magnetic-resonance spectroscopy. J Magn Reson B 107:242-251
Kruse B, Barker PB, van Zijl PC, Duyn JH, Moonen CT, Moser HW (1994) Multislice proton magnetic resonance spectroscopic imaging in X-linked adrenoleukodystrophy. Ann Neurol 36:595-608
Kruse B, Hanefeld F, Christen HJ, Bruhn H, Michaelis T, Hanicke W et al (1993) Alterations of brain metabolites in metachromatic leukodystrophy as detected by localized proton magnetic resonance spectroscopy in vivo. J Neurol 241:68-74
Leutner C, Layer G, Zierz S, Solymosi L, Dewes W, Reiser M (1994) Cerebral MR in ophthalmoplegia plus. AJNR Am J Neuroradiol 15:681-687
Martin E, Capone A, Schneider J, Hennig J, Thiel T (2001) Absence of N-acetylaspartate in the human brain: impact on neurospectroscopy? Ann Neurol 49:518-521
Matthews PM, Andermann F, Silver K, Karpati G, Arnold DL (1993) Proton MR spectroscopic characterization of differences in regional brain metabolic abnormalities in mitochondrial encephalomyopathies. Neurology 43:2484-2490 Matthews PM, de Stefano N, Narayanan S, Francis GS, Wolinsky JS, Antel JP et al (1998) Putting magnetic resonance spectroscopy studies in context: axonal damage and dis-
ability in multiple sclerosis. Semin Neurol 18:327-336 Nakai A, Goto Y, Fujisawa K, Shigematsu Y, Kikawa Y, Konishi Y
et al (1994) Diffuse leukodystrophy with a large-scale mitochondrial DNA deletion. Lancet 343:1397-1398
Narayana PA, Doyle TJ, Lai D, Wolinsky JS (1998) Serial proton magnetic resonance spectroscopic imaging, contrast-
enhanced magnetic resonance imaging, and quantitative lesion volumetry in multiple sclerosis. Ann Neurol 43:5671
Petroff OA, Graham GD, Blamire AM, al-Rayess M, Rothman DL, Fayad PB et al (1992) Spectroscopic imaging of stroke in humans: histopathology correlates of spectral changes. Neurology 42:1349-1354
Pietz J, Lutz T, Zwygart K, Hoffmann GF, Ebinger F, Boesch C et al (2003) Phenylalanine can be detected in brain tissue of healthy subjects by 1H magnetic resonance spectroscopy. J Inherit Metab Dis 26:683-692
Schiffmann R, van der Knaap MS (2004) The latest on leukodystrophies. Curr Opin Neurol 17:187-192
Schulze A (2003) Creatine deficiency syndromes. Mol Cell Biochem 244:143-150
Stockler S, Holzbach U, Hanefeld F, Marquardt I, Helms G, Requart M et al (1994) Creatine deficiency in the brain: a new, treatable inborn error of metabolism. Pediatr Res 36:409-413
Sylvain M, Arnold DL, Scriver CR, Schreiber R, Shevell MI (1994) Magnetic resonance spectroscopy in Neimann-Pick disease type C: correlation with diagnosis and clinical responce to cholestyramine and lovastatin. Pediatric Neurology 10:228-232
Tedeschi G, Schiffmann R, Barton NW, Shih HH, Gospe SMJ, Brady RO et al (1995) Proton magnetic resonance spectroscopic imaging in childhood ataxia with diffuse central nervous system hypomyelination. Neurology 45:1526-1532 Tzika AA, Ball WS Jr, Vigneron DB, Dunn RS, Nelson SJ, Kirks DR (1993) Childhood adrenoleukodystrophy: assessment
with proton MR spectroscopy. Radiology 189:467-480 Van der Knaap MS (2001) Magnetic resonance in childhood
white-matter disorders. Dev Med Child Neurol 43:705-712 Van der Knaap MS, Valk J, de Neeling N, Nauta JJ (1991) Pattern recognition in magnetic resonance imaging of white matter disorders in children and young adults. Neuroradi-
ology 33:478-493
Van der Knaap MS, Barth PG, Stroink H, van Nieuwenhuizen O, Arts WF, Hoogenraad F et al (1995) Leukoencephalopathy with swelling and a discrepantly mild clinical course in eight children. Ann Neurol 37:324-334
Van der Knaap MS, Barth PG, Gabreels FJ, Franzoni E, Begeer JH, Stroink H et al (1997) A new leukoencephalopathy with vanishing white matter. Neurology 48:845-855
Van der Knaap MS, Wevers RA, Struys EA, Verhoeven NM, Pouwels PJ, Engelke UF et al (1999) Leukoencephalopathy associated with a disturbance in the metabolism of polyols. Ann Neurol 46:925-928

Conventional MRI Techniques in Multiple Sclerosis |
209 |
Demyelinating Diseases