
- •Preface
- •Imaging Microscopic Features
- •Measuring the Crystal Structure
- •References
- •Contents
- •1.4 Simulating the Effects of Elastic Scattering: Monte Carlo Calculations
- •What Are the Main Features of the Beam Electron Interaction Volume?
- •How Does the Interaction Volume Change with Composition?
- •How Does the Interaction Volume Change with Incident Beam Energy?
- •How Does the Interaction Volume Change with Specimen Tilt?
- •1.5 A Range Equation To Estimate the Size of the Interaction Volume
- •References
- •2: Backscattered Electrons
- •2.1 Origin
- •2.2.1 BSE Response to Specimen Composition (η vs. Atomic Number, Z)
- •SEM Image Contrast with BSE: “Atomic Number Contrast”
- •SEM Image Contrast: “BSE Topographic Contrast—Number Effects”
- •2.2.3 Angular Distribution of Backscattering
- •Beam Incident at an Acute Angle to the Specimen Surface (Specimen Tilt > 0°)
- •SEM Image Contrast: “BSE Topographic Contrast—Trajectory Effects”
- •2.2.4 Spatial Distribution of Backscattering
- •Depth Distribution of Backscattering
- •Radial Distribution of Backscattered Electrons
- •2.3 Summary
- •References
- •3: Secondary Electrons
- •3.1 Origin
- •3.2 Energy Distribution
- •3.3 Escape Depth of Secondary Electrons
- •3.8 Spatial Characteristics of Secondary Electrons
- •References
- •4: X-Rays
- •4.1 Overview
- •4.2 Characteristic X-Rays
- •4.2.1 Origin
- •4.2.2 Fluorescence Yield
- •4.2.3 X-Ray Families
- •4.2.4 X-Ray Nomenclature
- •4.2.6 Characteristic X-Ray Intensity
- •Isolated Atoms
- •X-Ray Production in Thin Foils
- •X-Ray Intensity Emitted from Thick, Solid Specimens
- •4.3 X-Ray Continuum (bremsstrahlung)
- •4.3.1 X-Ray Continuum Intensity
- •4.3.3 Range of X-ray Production
- •4.4 X-Ray Absorption
- •4.5 X-Ray Fluorescence
- •References
- •5.1 Electron Beam Parameters
- •5.2 Electron Optical Parameters
- •5.2.1 Beam Energy
- •Landing Energy
- •5.2.2 Beam Diameter
- •5.2.3 Beam Current
- •5.2.4 Beam Current Density
- •5.2.5 Beam Convergence Angle, α
- •5.2.6 Beam Solid Angle
- •5.2.7 Electron Optical Brightness, β
- •Brightness Equation
- •5.2.8 Focus
- •Astigmatism
- •5.3 SEM Imaging Modes
- •5.3.1 High Depth-of-Field Mode
- •5.3.2 High-Current Mode
- •5.3.3 Resolution Mode
- •5.3.4 Low-Voltage Mode
- •5.4 Electron Detectors
- •5.4.1 Important Properties of BSE and SE for Detector Design and Operation
- •Abundance
- •Angular Distribution
- •Kinetic Energy Response
- •5.4.2 Detector Characteristics
- •Angular Measures for Electron Detectors
- •Elevation (Take-Off) Angle, ψ, and Azimuthal Angle, ζ
- •Solid Angle, Ω
- •Energy Response
- •Bandwidth
- •5.4.3 Common Types of Electron Detectors
- •Backscattered Electrons
- •Passive Detectors
- •Scintillation Detectors
- •Semiconductor BSE Detectors
- •5.4.4 Secondary Electron Detectors
- •Everhart–Thornley Detector
- •Through-the-Lens (TTL) Electron Detectors
- •TTL SE Detector
- •TTL BSE Detector
- •Measuring the DQE: BSE Semiconductor Detector
- •References
- •6: Image Formation
- •6.1 Image Construction by Scanning Action
- •6.2 Magnification
- •6.3 Making Dimensional Measurements With the SEM: How Big Is That Feature?
- •Using a Calibrated Structure in ImageJ-Fiji
- •6.4 Image Defects
- •6.4.1 Projection Distortion (Foreshortening)
- •6.4.2 Image Defocusing (Blurring)
- •6.5 Making Measurements on Surfaces With Arbitrary Topography: Stereomicroscopy
- •6.5.1 Qualitative Stereomicroscopy
- •Fixed beam, Specimen Position Altered
- •Fixed Specimen, Beam Incidence Angle Changed
- •6.5.2 Quantitative Stereomicroscopy
- •Measuring a Simple Vertical Displacement
- •References
- •7: SEM Image Interpretation
- •7.1 Information in SEM Images
- •7.2.2 Calculating Atomic Number Contrast
- •Establishing a Robust Light-Optical Analogy
- •Getting It Wrong: Breaking the Light-Optical Analogy of the Everhart–Thornley (Positive Bias) Detector
- •Deconstructing the SEM/E–T Image of Topography
- •SUM Mode (A + B)
- •DIFFERENCE Mode (A−B)
- •References
- •References
- •9: Image Defects
- •9.1 Charging
- •9.1.1 What Is Specimen Charging?
- •9.1.3 Techniques to Control Charging Artifacts (High Vacuum Instruments)
- •Observing Uncoated Specimens
- •Coating an Insulating Specimen for Charge Dissipation
- •Choosing the Coating for Imaging Morphology
- •9.2 Radiation Damage
- •9.3 Contamination
- •References
- •10: High Resolution Imaging
- •10.2 Instrumentation Considerations
- •10.4.1 SE Range Effects Produce Bright Edges (Isolated Edges)
- •10.4.4 Too Much of a Good Thing: The Bright Edge Effect Hinders Locating the True Position of an Edge for Critical Dimension Metrology
- •10.5.1 Beam Energy Strategies
- •Low Beam Energy Strategy
- •High Beam Energy Strategy
- •Making More SE1: Apply a Thin High-δ Metal Coating
- •Making Fewer BSEs, SE2, and SE3 by Eliminating Bulk Scattering From the Substrate
- •10.6 Factors That Hinder Achieving High Resolution
- •10.6.2 Pathological Specimen Behavior
- •Contamination
- •Instabilities
- •References
- •11: Low Beam Energy SEM
- •11.3 Selecting the Beam Energy to Control the Spatial Sampling of Imaging Signals
- •11.3.1 Low Beam Energy for High Lateral Resolution SEM
- •11.3.2 Low Beam Energy for High Depth Resolution SEM
- •11.3.3 Extremely Low Beam Energy Imaging
- •References
- •12.1.1 Stable Electron Source Operation
- •12.1.2 Maintaining Beam Integrity
- •12.1.4 Minimizing Contamination
- •12.3.1 Control of Specimen Charging
- •12.5 VPSEM Image Resolution
- •References
- •13: ImageJ and Fiji
- •13.1 The ImageJ Universe
- •13.2 Fiji
- •13.3 Plugins
- •13.4 Where to Learn More
- •References
- •14: SEM Imaging Checklist
- •14.1.1 Conducting or Semiconducting Specimens
- •14.1.2 Insulating Specimens
- •14.2 Electron Signals Available
- •14.2.1 Beam Electron Range
- •14.2.2 Backscattered Electrons
- •14.2.3 Secondary Electrons
- •14.3 Selecting the Electron Detector
- •14.3.2 Backscattered Electron Detectors
- •14.3.3 “Through-the-Lens” Detectors
- •14.4 Selecting the Beam Energy for SEM Imaging
- •14.4.4 High Resolution SEM Imaging
- •Strategy 1
- •Strategy 2
- •14.5 Selecting the Beam Current
- •14.5.1 High Resolution Imaging
- •14.5.2 Low Contrast Features Require High Beam Current and/or Long Frame Time to Establish Visibility
- •14.6 Image Presentation
- •14.6.1 “Live” Display Adjustments
- •14.6.2 Post-Collection Processing
- •14.7 Image Interpretation
- •14.7.1 Observer’s Point of View
- •14.7.3 Contrast Encoding
- •14.8.1 VPSEM Advantages
- •14.8.2 VPSEM Disadvantages
- •15: SEM Case Studies
- •15.1 Case Study: How High Is That Feature Relative to Another?
- •15.2 Revealing Shallow Surface Relief
- •16.1.2 Minor Artifacts: The Si-Escape Peak
- •16.1.3 Minor Artifacts: Coincidence Peaks
- •16.1.4 Minor Artifacts: Si Absorption Edge and Si Internal Fluorescence Peak
- •16.2 “Best Practices” for Electron-Excited EDS Operation
- •16.2.1 Operation of the EDS System
- •Choosing the EDS Time Constant (Resolution and Throughput)
- •Choosing the Solid Angle of the EDS
- •Selecting a Beam Current for an Acceptable Level of System Dead-Time
- •16.3.1 Detector Geometry
- •16.3.2 Process Time
- •16.3.3 Optimal Working Distance
- •16.3.4 Detector Orientation
- •16.3.5 Count Rate Linearity
- •16.3.6 Energy Calibration Linearity
- •16.3.7 Other Items
- •16.3.8 Setting Up a Quality Control Program
- •Using the QC Tools Within DTSA-II
- •Creating a QC Project
- •Linearity of Output Count Rate with Live-Time Dose
- •Resolution and Peak Position Stability with Count Rate
- •Solid Angle for Low X-ray Flux
- •Maximizing Throughput at Moderate Resolution
- •References
- •17: DTSA-II EDS Software
- •17.1 Getting Started With NIST DTSA-II
- •17.1.1 Motivation
- •17.1.2 Platform
- •17.1.3 Overview
- •17.1.4 Design
- •Simulation
- •Quantification
- •Experiment Design
- •Modeled Detectors (. Fig. 17.1)
- •Window Type (. Fig. 17.2)
- •The Optimal Working Distance (. Figs. 17.3 and 17.4)
- •Elevation Angle
- •Sample-to-Detector Distance
- •Detector Area
- •Crystal Thickness
- •Number of Channels, Energy Scale, and Zero Offset
- •Resolution at Mn Kα (Approximate)
- •Azimuthal Angle
- •Gold Layer, Aluminum Layer, Nickel Layer
- •Dead Layer
- •Zero Strobe Discriminator (. Figs. 17.7 and 17.8)
- •Material Editor Dialog (. Figs. 17.9, 17.10, 17.11, 17.12, 17.13, and 17.14)
- •17.2.1 Introduction
- •17.2.2 Monte Carlo Simulation
- •17.2.4 Optional Tables
- •References
- •18: Qualitative Elemental Analysis by Energy Dispersive X-Ray Spectrometry
- •18.1 Quality Assurance Issues for Qualitative Analysis: EDS Calibration
- •18.2 Principles of Qualitative EDS Analysis
- •Exciting Characteristic X-Rays
- •Fluorescence Yield
- •X-ray Absorption
- •Si Escape Peak
- •Coincidence Peaks
- •18.3 Performing Manual Qualitative Analysis
- •Beam Energy
- •Choosing the EDS Resolution (Detector Time Constant)
- •Obtaining Adequate Counts
- •18.4.1 Employ the Available Software Tools
- •18.4.3 Lower Photon Energy Region
- •18.4.5 Checking Your Work
- •18.5 A Worked Example of Manual Peak Identification
- •References
- •19.1 What Is a k-ratio?
- •19.3 Sets of k-ratios
- •19.5 The Analytical Total
- •19.6 Normalization
- •19.7.1 Oxygen by Assumed Stoichiometry
- •19.7.3 Element by Difference
- •19.8 Ways of Reporting Composition
- •19.8.1 Mass Fraction
- •19.8.2 Atomic Fraction
- •19.8.3 Stoichiometry
- •19.8.4 Oxide Fractions
- •Example Calculations
- •19.9 The Accuracy of Quantitative Electron-Excited X-ray Microanalysis
- •19.9.1 Standards-Based k-ratio Protocol
- •19.9.2 “Standardless Analysis”
- •19.10 Appendix
- •19.10.1 The Need for Matrix Corrections To Achieve Quantitative Analysis
- •19.10.2 The Physical Origin of Matrix Effects
- •19.10.3 ZAF Factors in Microanalysis
- •X-ray Generation With Depth, φ(ρz)
- •X-ray Absorption Effect, A
- •X-ray Fluorescence, F
- •References
- •20.2 Instrumentation Requirements
- •20.2.1 Choosing the EDS Parameters
- •EDS Spectrum Channel Energy Width and Spectrum Energy Span
- •EDS Time Constant (Resolution and Throughput)
- •EDS Calibration
- •EDS Solid Angle
- •20.2.2 Choosing the Beam Energy, E0
- •20.2.3 Measuring the Beam Current
- •20.2.4 Choosing the Beam Current
- •Optimizing Analysis Strategy
- •20.3.4 Ba-Ti Interference in BaTiSi3O9
- •20.4 The Need for an Iterative Qualitative and Quantitative Analysis Strategy
- •20.4.2 Analysis of a Stainless Steel
- •20.5 Is the Specimen Homogeneous?
- •20.6 Beam-Sensitive Specimens
- •20.6.1 Alkali Element Migration
- •20.6.2 Materials Subject to Mass Loss During Electron Bombardment—the Marshall-Hall Method
- •Thin Section Analysis
- •Bulk Biological and Organic Specimens
- •References
- •21: Trace Analysis by SEM/EDS
- •21.1 Limits of Detection for SEM/EDS Microanalysis
- •21.2.1 Estimating CDL from a Trace or Minor Constituent from Measuring a Known Standard
- •21.2.2 Estimating CDL After Determination of a Minor or Trace Constituent with Severe Peak Interference from a Major Constituent
- •21.3 Measurements of Trace Constituents by Electron-Excited Energy Dispersive X-ray Spectrometry
- •The Inevitable Physics of Remote Excitation Within the Specimen: Secondary Fluorescence Beyond the Electron Interaction Volume
- •Simulation of Long-Range Secondary X-ray Fluorescence
- •NIST DTSA II Simulation: Vertical Interface Between Two Regions of Different Composition in a Flat Bulk Target
- •NIST DTSA II Simulation: Cubic Particle Embedded in a Bulk Matrix
- •21.5 Summary
- •References
- •22.1.2 Low Beam Energy Analysis Range
- •22.2 Advantage of Low Beam Energy X-Ray Microanalysis
- •22.2.1 Improved Spatial Resolution
- •22.3 Challenges and Limitations of Low Beam Energy X-Ray Microanalysis
- •22.3.1 Reduced Access to Elements
- •22.3.3 At Low Beam Energy, Almost Everything Is Found To Be Layered
- •Analysis of Surface Contamination
- •References
- •23: Analysis of Specimens with Special Geometry: Irregular Bulk Objects and Particles
- •23.2.1 No Chemical Etching
- •23.3 Consequences of Attempting Analysis of Bulk Materials With Rough Surfaces
- •23.4.1 The Raw Analytical Total
- •23.4.2 The Shape of the EDS Spectrum
- •23.5 Best Practices for Analysis of Rough Bulk Samples
- •23.6 Particle Analysis
- •Particle Sample Preparation: Bulk Substrate
- •The Importance of Beam Placement
- •Overscanning
- •“Particle Mass Effect”
- •“Particle Absorption Effect”
- •The Analytical Total Reveals the Impact of Particle Effects
- •Does Overscanning Help?
- •23.6.6 Peak-to-Background (P/B) Method
- •Specimen Geometry Severely Affects the k-ratio, but Not the P/B
- •Using the P/B Correspondence
- •23.7 Summary
- •References
- •24: Compositional Mapping
- •24.2 X-Ray Spectrum Imaging
- •24.2.1 Utilizing XSI Datacubes
- •24.2.2 Derived Spectra
- •SUM Spectrum
- •MAXIMUM PIXEL Spectrum
- •24.3 Quantitative Compositional Mapping
- •24.4 Strategy for XSI Elemental Mapping Data Collection
- •24.4.1 Choosing the EDS Dead-Time
- •24.4.2 Choosing the Pixel Density
- •24.4.3 Choosing the Pixel Dwell Time
- •“Flash Mapping”
- •High Count Mapping
- •References
- •25.1 Gas Scattering Effects in the VPSEM
- •25.1.1 Why Doesn’t the EDS Collimator Exclude the Remote Skirt X-Rays?
- •25.2 What Can Be Done To Minimize gas Scattering in VPSEM?
- •25.2.2 Favorable Sample Characteristics
- •Particle Analysis
- •25.2.3 Unfavorable Sample Characteristics
- •References
- •26.1 Instrumentation
- •26.1.2 EDS Detector
- •26.1.3 Probe Current Measurement Device
- •Direct Measurement: Using a Faraday Cup and Picoammeter
- •A Faraday Cup
- •Electrically Isolated Stage
- •Indirect Measurement: Using a Calibration Spectrum
- •26.1.4 Conductive Coating
- •26.2 Sample Preparation
- •26.2.1 Standard Materials
- •26.2.2 Peak Reference Materials
- •26.3 Initial Set-Up
- •26.3.1 Calibrating the EDS Detector
- •Selecting a Pulse Process Time Constant
- •Energy Calibration
- •Quality Control
- •Sample Orientation
- •Detector Position
- •Probe Current
- •26.4 Collecting Data
- •26.4.1 Exploratory Spectrum
- •26.4.2 Experiment Optimization
- •26.4.3 Selecting Standards
- •26.4.4 Reference Spectra
- •26.4.5 Collecting Standards
- •26.4.6 Collecting Peak-Fitting References
- •26.5 Data Analysis
- •26.5.2 Quantification
- •26.6 Quality Check
- •Reference
- •27.2 Case Study: Aluminum Wire Failures in Residential Wiring
- •References
- •28: Cathodoluminescence
- •28.1 Origin
- •28.2 Measuring Cathodoluminescence
- •28.3 Applications of CL
- •28.3.1 Geology
- •Carbonado Diamond
- •Ancient Impact Zircons
- •28.3.2 Materials Science
- •Semiconductors
- •Lead-Acid Battery Plate Reactions
- •28.3.3 Organic Compounds
- •References
- •29.1.1 Single Crystals
- •29.1.2 Polycrystalline Materials
- •29.1.3 Conditions for Detecting Electron Channeling Contrast
- •Specimen Preparation
- •Instrument Conditions
- •29.2.1 Origin of EBSD Patterns
- •29.2.2 Cameras for EBSD Pattern Detection
- •29.2.3 EBSD Spatial Resolution
- •29.2.5 Steps in Typical EBSD Measurements
- •Sample Preparation for EBSD
- •Align Sample in the SEM
- •Check for EBSD Patterns
- •Adjust SEM and Select EBSD Map Parameters
- •Run the Automated Map
- •29.2.6 Display of the Acquired Data
- •29.2.7 Other Map Components
- •29.2.10 Application Example
- •Application of EBSD To Understand Meteorite Formation
- •29.2.11 Summary
- •Specimen Considerations
- •EBSD Detector
- •Selection of Candidate Crystallographic Phases
- •Microscope Operating Conditions and Pattern Optimization
- •Selection of EBSD Acquisition Parameters
- •Collect the Orientation Map
- •References
- •30.1 Introduction
- •30.2 Ion–Solid Interactions
- •30.3 Focused Ion Beam Systems
- •30.5 Preparation of Samples for SEM
- •30.5.1 Cross-Section Preparation
- •30.5.2 FIB Sample Preparation for 3D Techniques and Imaging
- •30.6 Summary
- •References
- •31: Ion Beam Microscopy
- •31.1 What Is So Useful About Ions?
- •31.2 Generating Ion Beams
- •31.3 Signal Generation in the HIM
- •31.5 Patterning with Ion Beams
- •31.7 Chemical Microanalysis with Ion Beams
- •References
- •Appendix
- •A Database of Electron–Solid Interactions
- •A Database of Electron–Solid Interactions
- •Introduction
- •Backscattered Electrons
- •Secondary Yields
- •Stopping Powers
- •X-ray Ionization Cross Sections
- •Conclusions
- •References
- •Index
- •Reference List
- •Index
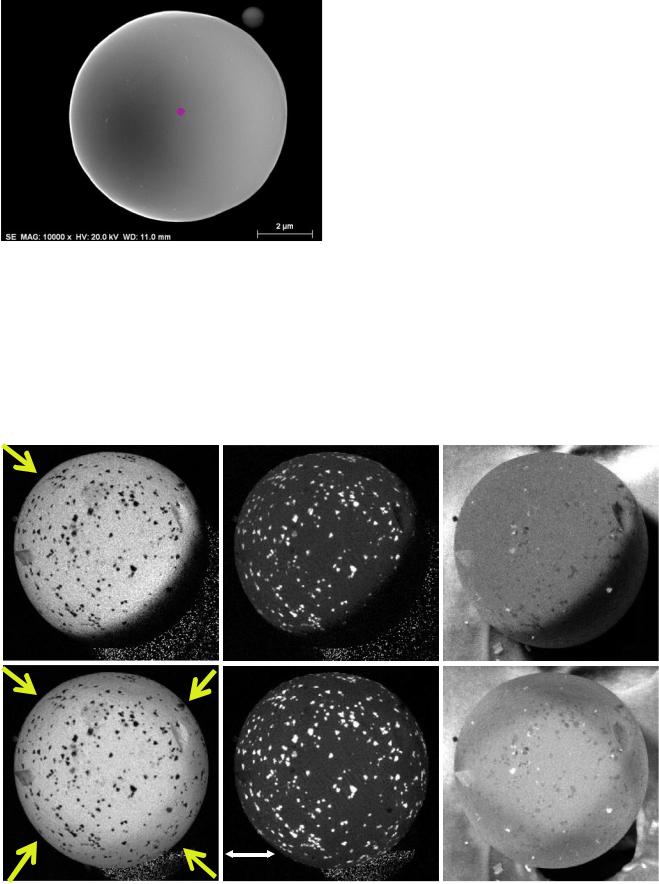
23.6 · Particle Analysis |
|
403 |
|
|
23 |
||||||
|
|
|
|
|
|
||||||
|
|
|
|
|
detector (SDD)-EDS inevitably shows the shadowing cre- |
||||||
|
|
|
|
|
ated by specimen geometry, such shadowing can be greatly |
||||||
|
|
|
|
|
diminished by collecting the XSI with an array of SDD-EDS |
||||||
|
|
|
|
|
detectors placed symmetrically around the specimen, as |
||||||
|
|
|
|
|
shown in . Fig. 23.29. |
|
|
||||
|
|
|
|
|
23.6.4\ Particle Geometry Factors |
|
|
||||
|
|
|
|
|
Influencing Quantitative Analysis |
|
|
||||
|
|
|
|
|
of Particles |
|
|
||||
|
|
|
|
|
|
|
|||||
|
|
|
|
|
There are two principal “particle geometry” effects: the “par- |
||||||
|
|
|
|
|
|||||||
|
|
|
|
|
ticle mass effect” and the “particle absorption effect” (Small |
||||||
|
|
|
|
|
et al. 1978, 1979). |
|
|
||||
|
|
|
|
|
|
|
|||||
. Fig. 23.28 Beam placement strategies for particle analysis: static |
“Particle Mass Effect” |
|
|
||||||||
The penetration of beam electrons through the side and bot- |
|||||||||||
point beam placed at particle center, overscanning with a scan field |
|||||||||||
that brackets the particle (yellow box), overscanning within the particle |
tom of a particle reduces the X-ray production compared to |
||||||||||
(green box) |
a flat bulk target, creating the so called “particle mass effect.” |
||||||||||
|
|
|
|
|
|||||||
as the inclusions, for subsequent analysis. All of this infor- |
. Figure 23.30 shows the intensity of Fe K-L2,3 produced as a |
||||||||||
function of sphere diameter for spherical particles of NIST |
|||||||||||
mation would be lost if these particles were simply over- |
SRM (K411), the composition of which is listed |
in |
|||||||||
scanned. While the XSI collected with a single silicon drift |
. Table 23.3, as calculated with the Monte Carlo simulation |
Comparison of mapping with a single SDD-EDS and an array of SDD-EDS
Ni |
Ti |
Total |
Ni 50 µm |
Ti |
Total |
. Fig. 23.29 Comparison of X-ray spectrum imaging with a single EDS and with an array of four EDS detectors; note reduction in shadowing
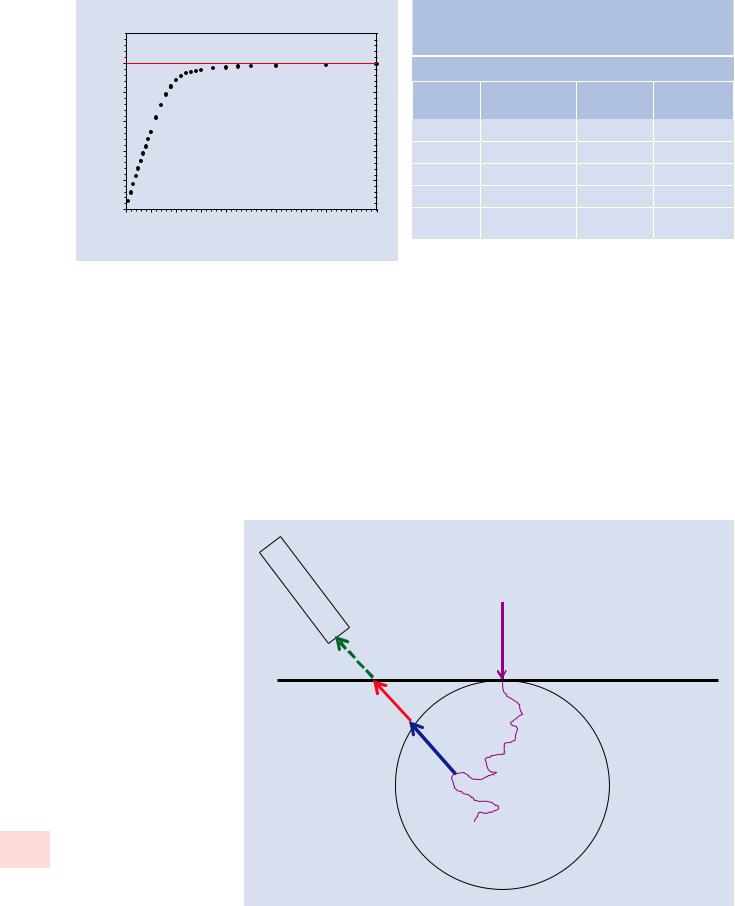
\404 Chapter 23 · Analysis of Specimens with Special Geometry: Irregular Bulk Objects and Particles
Fe k-ratio (bulk K411)
Monte Carlo Simulation Fe in K411 Spheres, E0 = 20 keV
1.2
1.0
0.8
0.6
0.4
0.2
0.0
0 |
1 |
2 |
3 |
4 |
5 |
6 |
7 |
8 |
9 |
10 |
|
|
|
Sphere diameter (µm) |
|
|
|
. Table 23.3 Composition of K411 glass and analysis of flat, bulk target at E0 = 20 keV (Standards: Mg, Si, CaF2, Fe; oxygen by stoichiometry)
NIST SRM 470 certificate values
Element |
Mass |
Analysis |
Relative |
|
concentration |
|
error (%) |
O |
0.4236 |
0.4192 |
−1.0 |
Mg |
0.0885 |
0.0870 |
−1.7 |
Si |
0.2538 |
0.2512 |
−1.0 |
Ca |
0.1106 |
0.1082 |
−2.2 |
Fe |
0.1121 |
0.1134 |
1.1 |
. Fig. 23.30 Monte Carlo calculation of the emission of Fe K-L2,3 from K411 spheres of various diameters at E0 = 20 keV. The intensity is normalized by the Fe K-L2,3 intensity calculated for a flat bulk target of K411
for an incident beam energy of 20 keV. For this plot, the Fe K-L2,3 intensity emitted from the particle has been divided by the intensity calculated for a flat bulk K411 target to determine the k-ratio. Note that as the particle diameter increases from zero, the Fe K-L2,3 k-ratio increases and asymptotically approaches unity (i.e., equivalent to the flat bulk target) for a diameter of approximately 10 μm. Thus, for the case K411 at E0 = 20 keV, bulk behavior for Fe K-L2,3 (6.400 keV) is
observed for spherical particles with diameters of 10 μm and greater.
“Particle Absorption Effect”
The surface curvature of a particle can reduce the absorption path to the detector compared to the interaction volume in a flat bulk target, as shown schematically in . Fig. 23.31. Since X-ray absorption depends exponentially on the absorption path length, surface curvature can significantly modify the measured intensity, creating the “particle absorption effect.” The magnitude of the particle absorption effect depends strongly on the energy of the characteristic photons involved. For the example shown in . Fig. 23.32, the Fe K-L2,3
. Fig. 23.31 Schematic illustration of the difference in the X-ray absorption path length in a particle and in a flat bulk target of the same material
X |
|
|
- |
Incident |
|
ray |
|
|
detecto |
beam |
|
|
|
|
r |
|
|
Path through vacuum |
|
|
Additional |
|
|
absorption path |
Absorption |
|
in flat bulk target |
path |
|
|
in particle |
Electron |
|
|
trajectory |
23
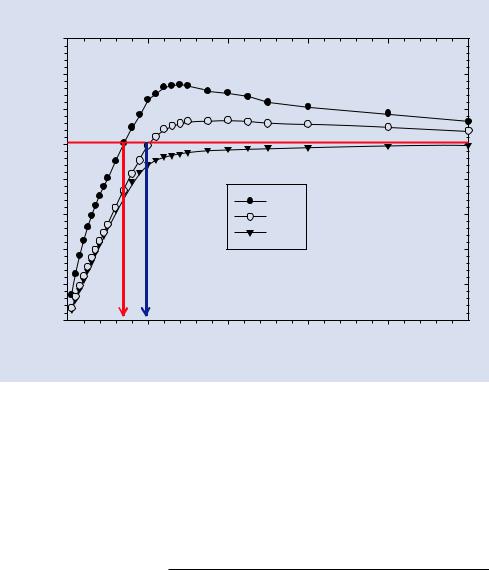
23.6 · Particle Analysis
. Fig. 23.32 Emission of Fe
K-L2,3, O K-L2 and Mg K-L2,3 (normalized to O K-L2 and Mg K-L2,3 from bulk K411); note excursion
in the O K-L2,3 and Mg K-L2,3 above unity (i.e., exceeding the
emission from a flat, bulk target)
k-ratio (K411 bulk)
405 |
|
23 |
|
|
|
Monte Carlo calculations of K411 Spheres (E0 = 20 keV)
1.6
1.8
1.2
1.0
0.8 |
|
|
|
|
|
|
|
|
O |
|
|
0.6 |
|
|
Mg |
|
|
|
|
|
FeK |
|
|
0.4 |
|
|
|
|
|
0.2 |
|
|
|
|
|
0.0 |
|
|
|
|
|
0 |
2 |
4 |
6 |
8 |
10 |
Diameter (micrometers)
radiation at 6.40 keV is sufficiently energetic that it undergoes relatively little absorption in the bulk case so that the modification of the absorption path length by particle surface curvature produces a negligible effect. However, the lower energy characteristic photons such as O K-L2 (0.525 keV) and Mg K-L2,3 (1.254 keV) in K411 suffer significant absorption in a flat bulk target, so that when these photons are generated in a spherical particle, the reduced absorption path in the direction toward the EDS leads to an increase in X-ray emission compared to a flat bulk target.
. Figure 23.32 shows that as the particle diameter increases
from zero, the k-ratios for O K-L2 and Mg K-L2,3 initially increase similarly to FeKα as a result of the particle mass
effect. However, for larger particles the reduced absorption path of the curved particle surface causes the emitted O K-L2 k-ratio to actually exceed unity (i.e., higher emission than bulk behavior) for a particle diameter of 1.6 μm, reaching a maximum of 1.35 relative to bulk at a particle diameter
of 2.8 μm. For Mg K-L2,3, the emission exceeds unity for a particle diameter of 2 μm and reaches a maximum of 1.17 at
a diameter of 3.0 μm. For particle diameters beyond the
intensity maxima, the O K-L2 and Mg K-L2,3 k-ratios gradually decrease with increasing particle diameter, asymptoti-
cally approaching the equivalent of bulk behavior at 25 μm
diameter for O K-L2, and 18 μm diameter for Mg K-L2,3. Thus, for spherical particles of the K-411 composition mea-
sured with E0 = 20 keV, effectively bulk behavior is observed for all characteristic X-ray energies for particles with diameters greater than 25 μm for a beam position at the top center of the particle (detector take-off angle 40°). As demonstrated in . Fig. 23.23, deviations in the beam placement either toward the EDS or away have significant effects due to the
modification of the absorption path. Particle geometry and its impact on X-ray absorption must be considered when selecting beam locations on a particle for analysis. It is critical that the analyst always be aware of the position of the EDS detector relative to the X-ray source, as demonstrated in
. Fig. 23.24, to minimize the effects of particle geometry.
23.6.5\ Uncertainty in Quantitative Analysis
of Particles
Quantitative analysis of particles is performed by following the same k-ratio/matrix correction protocol used for flat, bulk specimens. However, it must be recognized that particle geometry modification of the interaction of beam electrons and the subsequent propagation of X-rays introduce factors which violate the fundamental assumption of the bulk quantification method, namely that the only reason the X-ray intensities measured in the target being analyzed are different from the standards is that the composition(s) is different. Thus, with the impact of the geometric factors, the analytical accuracy of the conventional k-ratio/matrix correction protocol is inevitably compromised. The critical question to consider is the degree to which the uncertainty budget is increased by the systematic error contribution of the particle effects.
The Analytical Total Reveals the Impact of Particle Effects
The analytical total is the sum of the calculated concentrations, including oxygen by stoichiometry if calculated.
. Table 23.3 shows the results of the analysis of K411 glass in the form of a flat, bulk target. The beam energy was
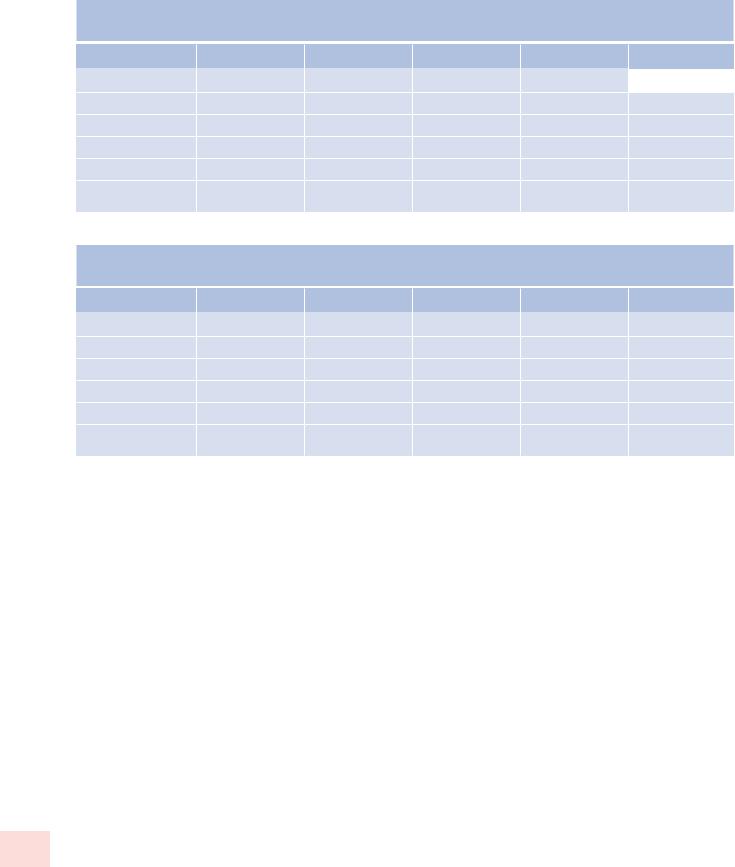
406\ Chapter 23 · Analysis of Specimens with Special Geometry: Irregular Bulk Objects and Particles
. Table 23.4 Analysis of a 1.3-μm-diameter spherical particle of K411 glass with fixed beam located at particle center (standards: Mg, Si,
CaF2, Fe; oxygen by stoichiometry)
Element |
SRM value |
Analysis |
Rel error (%) |
Normalized |
Rel error (%) |
|
|
|
|
|
|
O |
0.4236 |
0.1470 |
−65 |
0.4224 |
−0.3 |
Mg |
0.0885 |
0.0265 |
−70 |
0.0761 |
−14 |
Si |
0.2538 |
0.0884 |
−65 |
0.2541 |
+0.1 |
Ca |
0.1106 |
0.0370 |
−67 |
0.1064 |
−3.8 |
Fe |
0.1121 |
0.0491 |
−56 |
0.1410 |
+26 |
Raw analytical total |
0.3480 |
|
|
|
|
. Table 23.5 Analysis of a 6.1-μm-diameter spherical particle of K411 glass with fixed beam located at particle center (standards: Mg, Si,
Ca [K412 glass], Fe; oxygen by stoichiometry)
Element |
SRM value |
Analysis |
Rel error (%) |
Normalized |
Rel error (%) |
O |
0.4236 |
0.4748 |
+12 |
0.4353 |
+2.8 |
Mg |
0.0885 |
0.1110 |
+25 |
0.1018 |
+15 |
Si |
0.2538 |
0.2874 |
+13 |
0.2636 |
+3.9 |
Ca |
0.1106 |
0.1062 |
−4.0 |
0.0974 |
−12 |
Fe |
0.1121 |
0.1112 |
−0.8 |
0.1019 |
−9.1 |
Raw analytical total |
1.091 |
|
|
|
|
E0 = 20 keV, and the standards used pure elements Mg, Si, and Fe, while SRM 470 K412 glass was used as the Ca standard. The analytical total was 0.9789 (the sum of the SRM certificate values is 0.9886), and the relative errors were all well within the ±5 % error envelope, with the largest error at −2.2 % relative for Ca.
. Table 23.4 gives the results of applying the k-ratio/ matrix correction factor protocol to the analysis of a 1.3-μm-diameter spherical particle of K411 glass, with the EDS spectrum collected with the beam placed at the center of the particle image. The intensity of each elemental constituent has been measured relative to the same suite of standards: pure elements Mg, Si, and Fe, and CaF2 as the Ca standard. Oxygen has been calculated by the method of assumed stoichiometry. The analytical total is 0.3480, and the relative errors for the raw calculated concentrations are large and negative, for example, −70 % relative for Mg and −56 % relative for Fe. These large negative relative errors (a negative relative error indicates that the calculated concentration underestimates the true concentration) for both the low pho-
ton energy peaks (e.g., Mg K-L2,3 and Si K-L2,3) and the high photon energy peaks (e.g., Ca Kα and Fe Kα) are a result of
23 the particle mass effect reducing all X-ray intensities compared to bulk behavior. Clearly, these raw concentrations
have such large systematic errors as to offer no realistic meaning. To compensate for the mass effect and thus place the concentrations on a meaningful basis, internal normalization can be applied:
Cn (i) = Ci / ΣCi |
(23.3) |
|
\ |
Note that normalization is only useful if all constituents present in the analyzed volume are included in the total, including any such as oxygen that are calculated by assumed stoichiometry rather than measured directly. After normalization, the relative errors are reduced in magnitude, as given in . Table 23.4, but the values for Mg (−14 %) and Fe (+26 %) remain well outside the bulk analysis error histogram.
. Table 23.5 presents similar measurements and calculations for a 6.1-μm-diameter K411 particle for which the analytical total is 1.091. This particle diameter is sufficiently large so that the X-ray production for the higher energy photons,
Ca K-L2,3 and Fe K-L2,3, has nearly reached equivalence to the flat, bulk target, resulting in relative errors of −5 % or less.
For this particle size, the lower energy photon peaks, Mg
K-L2,3 and Si K-L2,3, are still strongly influenced by the particle absorption effect, causing relative errors that are large and
positive, since more of these low energy photons escape than