
- •Contents
- •1.1 Introduction
- •1.2 Selection of dental materials
- •1.3 Evaluation of materials
- •2.1 Introduction
- •2.2 Mechanical properties
- •2.3 Rheological properties
- •2.4 Thermal properties
- •2.5 Adhesion
- •2.6 Miscellaneous physical properties
- •2.7 Chemical properties
- •2.8 Biological properties
- •2.9 Suggested further reading
- •3.1 Introduction
- •3.2 Requirements of dental cast materials
- •3.3 Composition
- •3.4 Manipulation and setting characteristics
- •3.5 Properties of the set material
- •3.6 Applications
- •3.7 Advantages and disadvantages
- •3.8 Suggested further reading
- •4.1 Introduction
- •4.2 Requirements of wax-pattern materials
- •4.3 Composition of waxes
- •4.4 Properties of dental waxes
- •4.5 Applications
- •4.6 Suggested further reading
- •5.1 Introduction
- •5.2 Requirements of investments for alloy casting procedures
- •5.3 Available materials
- •5.4 Properties of investment materials
- •5.5 Applications
- •5.6 Suggested further reading
- •6.1 Introduction
- •6.2 Structure and properties of metals
- •6.3 Structure and properties of alloys
- •6.4 Cooling curves
- •6.5 Phase diagrams
- •6.6 Suggested further reading
- •7.1 Introduction
- •7.2 Pure gold fillings (cohesive gold)
- •7.3 Traditional casting gold alloys
- •7.4 Hardening heat treatments (theoretical considerations)
- •7.5 Heat treatments (practical considerations)
- •7.6 Alloys with noble metal content of at least 25% but less than 75%
- •7.7 Soldering and brazing materials for noble metals
- •7.8 Noble alloys for metal-bonded ceramic restorations
- •7.9 Biocompatibility
- •7.10 Suggested further reading
- •8.1 Introduction
- •8.2 Composition
- •8.3 Manipulation of base metal casting alloys
- •8.4 Properties
- •8.5 Comparison with casting gold alloys
- •8.6 Biocompatibility
- •8.7 Metals and alloys for implants
- •8.8 Suggested further reading
- •9.1 Introduction
- •9.2 Investment mould
- •9.3 Casting machines
- •9.4 Faults in castings
- •9.5 Suggested further reading
- •10.1 Introduction
- •10.2 Steel
- •10.3 Stainless steel
- •10.4 Stainless steel denture bases
- •10.5 Wires
- •10.6 Suggested further reading
- •11.1 Introduction
- •11.2 Composition of traditional dental porcelain
- •11.3 Compaction and firing
- •11.4 Properties of porcelain
- •11.5 Alumina inserts and aluminous porcelain
- •11.6 Sintered alumina core ceramics
- •11.7 Injection moulded and pressed ceramics
- •11.8 Cast glass and polycrystalline ceramics
- •11.9 CAD–CAM restorations
- •11.10 Porcelain veneers
- •11.11 Porcelain fused to metal (PFM)
- •11.12 Capillary technology
- •11.13 Bonded platinum foil
- •11.14 Suggested further reading
- •12.1 Introduction
- •12.2 Polymerisation
- •12.3 Physical changes occurring during polymerisation
- •12.4 Structure and properties
- •12.5 Methods of fabricating polymers
- •12.6 Suggested further reading
- •13.1 Introduction
- •13.2 Requirements of denture base polymers
- •13.3 Acrylic denture base materials
- •13.4 Modified acrylic materials
- •13.5 Alternative polymers
- •13.6 Suggested further reading
- •14.1 Introduction
- •14.2 Hard reline materials
- •14.3 Tissue conditioners
- •14.4 Temporary soft lining materials
- •14.5 Permanent soft lining materials
- •14.6 Self-administered relining materials
- •14.7 Suggested further reading
- •15.1 Introduction
- •15.2 Requirements
- •15.3 Available materials
- •15.4 Properties
- •15.5 Suggested further reading
- •16.1 Introduction
- •16.2 Classification of impression materials
- •16.3 Requirements
- •16.4 Clinical considerations
- •16.5 Suggested further reading
- •17.1 Introduction
- •17.2 Impression plaster
- •17.3 Impression compound
- •17.4 Impression waxes
- •18.1 Introduction
- •18.2 Reversible hydrocolloids (agar)
- •18.3 Irreversible hydrocolloids (alginates)
- •18.5 Modified alginates
- •18.6 Suggested further reading
- •19.1 Introduction
- •19.2 Polysulphides
- •19.3 Silicone rubbers (condensation curing)
- •19.4 Silicone rubbers (addition curing)
- •19.5 Polyethers
- •19.6 Comparison of the properties of elastomers
- •19.7 Suggested further reading
- •20.1 Introduction
- •20.2 Appearance
- •20.3 Rheological properties and setting characteristics
- •20.4 Chemical properties
- •20.5 Thermal properties
- •20.6 Mechanical properties
- •20.7 Adhesion
- •20.8 Biological properties
- •20.9 Historical
- •21.1 Introduction
- •21.2 Composition
- •21.3 Setting reactions
- •21.4 Properties
- •21.6 Manipulative variables
- •21.7 Suggested further reading
- •22.1 Introduction
- •22.2 Acrylic resins
- •22.3 Composite materials – introduction
- •22.4 Classification and composition of composites
- •22.5 Properties of composites
- •22.6 Fibre reinforcement of composite structures
- •22.7 Clinical handling notes for composites
- •22.8 Applications of composites
- •22.9 Suggested further reading
- •23.1 Introduction
- •23.2 Acid-etch systems for bonding to enamel
- •23.3 Applications of the acid-etch technique
- •23.4 Bonding to dentine – background
- •23.5 Dentine conditioning – the smear layer
- •23.6 Priming and bonding
- •23.7 Current concepts in dentine bonding – the hybrid layer
- •23.8 Classification of dentine bonding systems
- •23.9 Bonding to alloys, amalgam and ceramics
- •23.10 Bond strength and leakage measurements
- •23.11 Polymerizable luting agents
- •23.12 Suggested further reading
- •24.1 Introduction
- •24.2 Composition
- •24.3 Setting reaction
- •24.4 Properties
- •24.5 Cermets
- •24.6 Applications and clinical handling notes
- •24.7 Suggested further reading
- •25.1 Introduction
- •25.2 Composition and classification
- •25.3 Setting characteristics
- •25.4 Dimensional change and dimensional stability
- •25.5 Mechanical properties
- •25.6 Adhesive characteristics
- •25.7 Fluoride release
- •25.8 Clinical handling notes
- •25.9 Suggested further reading
- •26.1 Introduction
- •26.2 Requirements
- •26.3 Available materials
- •26.4 Properties
- •27.1 Introduction
- •27.2 Requirements of cavity lining materials
- •27.3 Requirements of Iuting materials
- •27.4 Requirements of endodontic cements
- •27.5 Requirements of orthodontic cements
- •27.6 Suggested further reading
- •28.1 Introduction
- •28.2 Zinc phosphate cements
- •28.3 Silicophosphate cements
- •28.4 Copper cements
- •28.5 Suggested further reading
- •29.1 Introduction
- •29.2 Zinc oxide/eugenol cements
- •29.3 Ortho-ethoxybenzoic acid (EBA) cements
- •29.4 Calcium hydroxide cements
- •29.5 Suggested further reading
- •30.1 Introduction
- •30.2 Polycarboxylate cements
- •30.3 Glass ionomer cements
- •30.4 Resin-modified glass ionomers and compomers
- •30.5 Suggested further reading
- •31.1 Introduction
- •31.2 Irrigants and lubricants
- •31.3 Intra-canal medicaments
- •31.4 Endodontic obturation materials
- •31.5 Historical materials
- •31.6 Contemporary materials
- •31.7 Clinical handling
- •31.8 Suggested further reading
- •Appendix 1
- •Index

Ceramics and Porcelain Fused to Metal (PFM) |
93 |
|
|
Table 11.2 Flexural strength values (MPa) of some dental ceramics.
Unreinforced porcelain |
70–120 |
Aluminous porcelain |
120–180 |
Cast glass ceramic |
100–150 |
Sintered alumina core ceramic |
400–600 |
Sintered alumina with zirconia |
800 |
Pressed glass with leucite |
120–180 |
Yttrium tetragonal zirconia polycrystals |
900–1200 |
Minimum for core ceramic (ISO Standard) |
100 |
Minimum for dentine/enamel ceramic (ISO |
50 |
Standard) |
|
|
|
around the ‘reinforcing’ material as illustrated in Fig. 11.2b.
Porcelain which contains alumina is referred to as aluminous porcelain and the alumina content is normally around 40%. Although aluminous porcelain has definite advantages in terms of mechanical properties, it is opaque and therefore can only be used to construct the inner core region of a porcelain crown. This is generally acceptable, since it is the inner region from which cracks propagate and which is therefore the area in need of reinforcement. A matched expansion veneering porcelain is baked onto the surface of the high alumina material to give the finished restoration. Flexural strength values of aluminous porcelains are typically in excess of 110 MPa compared to only about 80 MPa for unreinforced materials (Table 11.2).
11.6 Sintered alumina core ceramics
The use of alumina additions to strengthen porcelain has been taken a stage further with the introduction of sintered alumina cores. For one such
Fig. 11.2 Diagram illustrating how the propagation of a crack can be halted by a reinforcing particle. (a) Alumina particle acting as a crack stopper. (b) A crack propagating around a filler particle.
system (Inceram) the first stage in producing the restoration involves the formation of a duplicate die using a ‘special’ plaster. An alumina slip prepared from alumina powder and water is then painted on to the die. The moisture from the slip is absorbed by the plaster leaving a layer of alumina powder which should ideally be at least 0.5 mm thick. This is then sintered by firing at 1120ºC for 2 hours. The firing causes the die material to shrink, making the removal of the sintered alumina core quite easy. The outer surface of the core is painted with a slurry of a glass powder and firing at 1100ºC is carried out to liquefy the glass which flows to fill all the spaces between the sintered alumina particles. The glass used is a lanthanum aluminosilicate glass. The lanthanum reduces viscosity and assists infiltration. It also increases the refractive index of the glass and improves the translucency of the ceramic. After microblasting to remove gross excesses of glass a refiring at 960º is performed to ensure proper infiltration of the alumina with glass.
On completion of the core firing procedures, the dentine and enamel layers are built up in the traditional manner. The extremely high flexural strength of the sintered alumina system (Table 11.2) gives rise to some optimism that these materials may be suitable for the production of multiunit restorations which have previously required the use of cast-metal substructures. Spinel-based cores of magnesium aluminate have been used instead of alumina. These produce improved translucency but are not as strong as sintered alumina cores.
A further advance has been the development of a sintered alumina core containing significant quantities of zirconium oxide to achieve further strengthening and a reported flexural strength of

94 Chapter 11
up to 800 MPa. One limitation of this system is the relative opacity of the resulting core which may be difficult to mask with glass infiltration and may therefore limit the aesthetic qualities of the final restoration.
11.7 Injection moulded and pressed ceramics
These materials were first reported in 1983 for the production of all-ceramic, single, anterior or posterior crowns. The first commonly used commercial system based on this principle (Cerestore) involved the production of crown cores by injection moulding, potentially eliminating the need for the use of a platinum foil and improving marginal adaptation of the crown. The non-shrink properties are achieved by incorporating significant quantities of magnesium oxide into the ceramic frit. This reacts with the alumina during firing to form a mixed metal oxide, called a spinel. The spinel is less dense than the original mixture of oxides and its formation results in an expansion which compensates for firing shrinkage.
The technique for fabricating ceramic copings from this type of material involves the formation of a wax pattern on an epoxy resin die. A variation of the lost wax technique is used to maintain the correct shape and size in the ceramic material. The mixed material, containing magnesium and aluminium oxides, glass, kaolin, calcium stearate and wax is injected under pressure at 180ºC into the prepared mould. Firing is then carried out in a special furnace using controlled temperatures up to 1300ºC during which formation of the spinel occurs. Veneer porcelains are baked onto the surface of the coping to produce the finished crown.
It was hoped that these ceramic copings would offer the possibility of constructing all-ceramic crowns for molars with the attendant improvement in aesthetics which this would bring. The strength of the material is however, not much different from that of ordinary aluminous porcelain and the complexity of the process involving the need for special equipment has limited its use.
A further development of this idea of injection moulding involved the introduction of a material (IPS Empress) in which a molten feldspathic type glass is pressed into a pre-formed mould formed using a high temperature investment material and a wax pattern (see Section 9.2). After the molten
glass has been forced into the mould under pressure, it is allowed to cool under controlled conditions which allows reinforcing leucite crystals to form. The properties of the material are similar to those of the cast glass ceramics.
One of two methods can be used for characterization. For anterior crowns a layering technique is normally used to achieve the best possible appearance. A fully sized crown is first produced from a wax pattern. This structure is then ‘cut back’ in those areas where conventional layered porcelains are used to give optimal aesthetics to give adequate space for the veneering material. During the firing of the outer veneer layer of porcelain further strengthening of the pressed ceramic core may occur as the concentration of leucite crystallites increases.
For posterior crowns, inlays or labial veneers where either aesthetics are not considered so important or where layering would be impossible due to insufficient thickness, characterization is achieved by the application of surface stains.
The latest version of the pressed ceramic approach (IPS Empress 2) is based upon lithium disilicate ceramic. These materials can be fabricated to give material having a flexural strength approaching that of the sintered alumina core ceramics (300–400 MPa). The materials are recommended for the fabrication of 3-unit bridges (fixed partial dentures) to replace missing anterior or premolar teeth.
11.8 Cast glass and polycrystalline ceramics
The approach to casting ceramics is very similar to that used in pressing and moulding described in the previous section. The processes used here are essentially the same as those used in the lostwax technique used in alloy casting. Currently, systems falling within this category are used only for the manufacture of single crowns and are being replaced by the stronger sintered alumina and pressed glass systems.
Crowns are formed from wax patterns which are invested in a phosphate-bonded investment. The wax burn-out and heat soak of the investment is carried out at about 950ºC. The molten ceramic is cast centrifugally into the mould at around 1350ºC. This results in a transparent glass crown which is then heat treated in an oven at 1075ºC for 10 hours. This heat treatment or ceramming causes partial crystallization to form mica-like
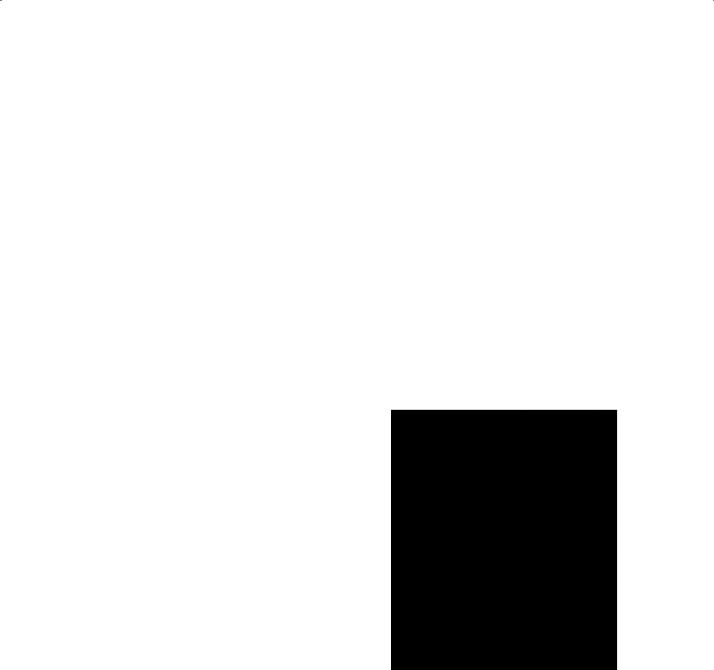
Ceramics and Porcelain Fused to Metal (PFM) |
95 |
|
|
crystals (containing K, Mg and Si oxides along with significant quantities of fluoride) which have a dual effect. They slightly reduce the translucency of the previously clear material and significantly increase the strength. Table 11.2 shows that the strength of these cast ceramics can match that of aluminous porcelain.
Colour matching is achieved by applying a series of the appropriate tinted porcelains to the surface and re-firing. This procedure may be circumvented with the development of new candidate materials in which the shading can be incorporated within the body of the crown. Further improvements in appearance are achieved by selecting the correct shade of luting cement. The surface glazes used to provide colour to cast glasses can produce a very realistic result. Unfortunately, if there is a need to undertake any adjustment of the shape of the crown they are rapidly removed, resulting in poor aesthetics unless the crown is re-glazed.
The technique of casting offers the ability to produce accurately fitting, all-ceramic crowns which may be strong enough for use in the posterior region without the need of an alloy substructure. The need for some specialist equipment may be considered a disadvantage.
Another material which is being introduced to dentistry is based upon yttrium tetragonal zirconia polycrystals (Y-TZP). Yttrium oxide is blended with zirconium oxide to generate a multiphase material known as partially stabilized zirconia. These restorations may be processed using casting procedures or may be milled from monolithic blocks of partially or fully sintered material (see CAD-CAM). Very high values of both flexural strength (900–1200 MPa) and fracture toughness have been reported for these Y-TZP ceramics. This is thought to be due to their polycrystalline structure and to a process of ‘transformation toughening’ caused by changes in crystal structure initiated by internal stresses. The transformations result in localized increases in volume which cause compressive stresses to be set up around crack tips. This reduces the likelihood of further propagation of the crack.
11.9 CAD–CAM restorations
tooth-coloured restorations. It involves recording an optical impression from which a restoration can be designed using a computer. The design details are then used to construct the restoration using a milling machine which cuts the desired shape from a monolithic block of ceramic under the control of the computer. Care is required during cavity preparation to ensure that there are no undercuts within the cavity. This technique is relatively flexible in terms of the shapes of restorations that can be made with modern milling technology. Complex 3 or 4 surface restorations are possible including the replacement of cusps. In addition it is possible to manufacture ceramic veneers using the latest generations of these systems (for example Cerec 3®1) as shown in Fig. 11.3. The optical impression is recorded using a miniature video camera which scans the prepared tooth for about 10 seconds. The prepared tooth
Fig. 11.3 CAD–CAM generated dental appliances. This shows a CAD–CAM equipment set up for manufacturing dental appliances and restorations at the chairside using computer generated images and computer controlled milling equipment. On the right of the figure is the control unit and the computer which is used for viewing the scanned images and on the left is the milling instrument in which the shapes are cut from a variety of materials. Figure reproduced with permission of www.planetcerec. com.
CAD-CAM stands for computer aided design – computer aided manufacture. This is a high-tech approach to providing patients with durable
1 Cerec 3 Sirona Dental Systems GmbH, Fabrikstrasse 31, 64625 Bensheim, Germany.
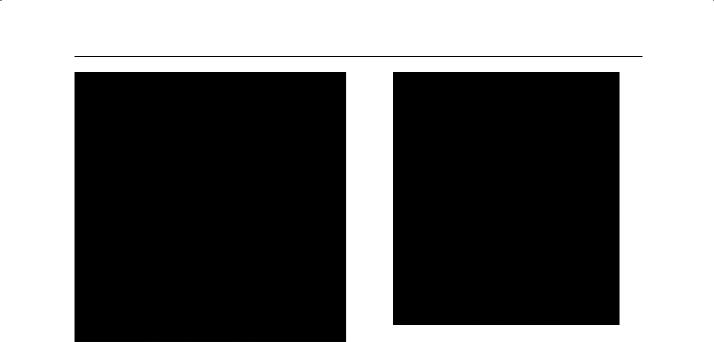
96 Chapter 11
Fig. 11.4 Milling of CAD–CAM restorations. Here we see the milling operation in progress. We can see that the blank has already been cut near to the final shape and following the completion of this process the dental restoration will be removed from the machine and will be ready to be cemented into the patient’s mouth. Figure reproduced with permission of www.planetcerec.com.
Fig. 11.5 Materials for CAD–CAM restorations. This shows blanks of materials which are used for milling the shapes required in the CAD–CAM milling machine shown in figure 11.3. The metal part of these blanks is held in the grips of the milling machine and the tooth coloured parts (ceramic or resin based) are milled to the correct shape. Figure reproduced with permission of www.planetcerec.com.
surface has to be clean and dry and is coated in an optically reflective powder to maximize image acquisition. For the sake of accuracy it is important that this layer is uniform and kept as thin as possible. An optical image of the opposing teeth can also be captured to allow the software to recreate the normal pattern of interdigitation between the teeth and hence to create an appropriate surface anatomy for the restorations concerned.
The design of the restoration on the computer screen takes between 10 and 25 minutes depending on the complexity of the restoration and the extent to which the software is asked to accommodate the patient’s occlusion. The milling process (Fig. 11.4) takes a further 5–10 minutes, again varying with the size and complexity of the procedure.
The restoration is tried in and adjusted if necessary. The try in is made easier for more complex shapes as there is a small spur on the finished restoration when if comes out of the milling machine which can be used as a handle while manipulating it. It is then normal to etch the ceramic fitting surface with hydrofluoric acid and then apply a silane coupling agent to aid retention before final cementation using a dual cured resin
cement. A final polishing can be performed in situ. Obviously it is not possible to provide a glazed ceramic surface with this technology. The beauty of the Cerec® system is that all of the stages of preparation and manufacture can be undertaken in the dental surgery; however it is a relatively timeconsuming process as a consequence.
An alternative to the chair side CAD–CAM approach is the use of a mechanical copy-milling technique in the laboratory. The clinical stages are identical to those for a conventional inlay. A pattern is produced in the laboratory and then a replica of that pattern produced in a ceramic using a copy-milling device. The commercially available systems are capable of reproducing both relatively intricate fitting surfaces and the occlusal surface of the inlay.
Materials used to form the ceramic block (Fig. 11.5) from which the restoration is cut include conventional feldspathic porcelains and glass ceramics. It has recently been shown that restorations can be cut from sintered alumina blocks which can be infiltrated with glass to form In-ceram-like CAD–CAM restorations. A variety of approuches to providing CAD-CAM restorations are now available to dentists. If a dentisk