
ДНК-нанотехнологии 1 введение и основные методы / 10.1021@acs.chemrev.0c00294
.pdf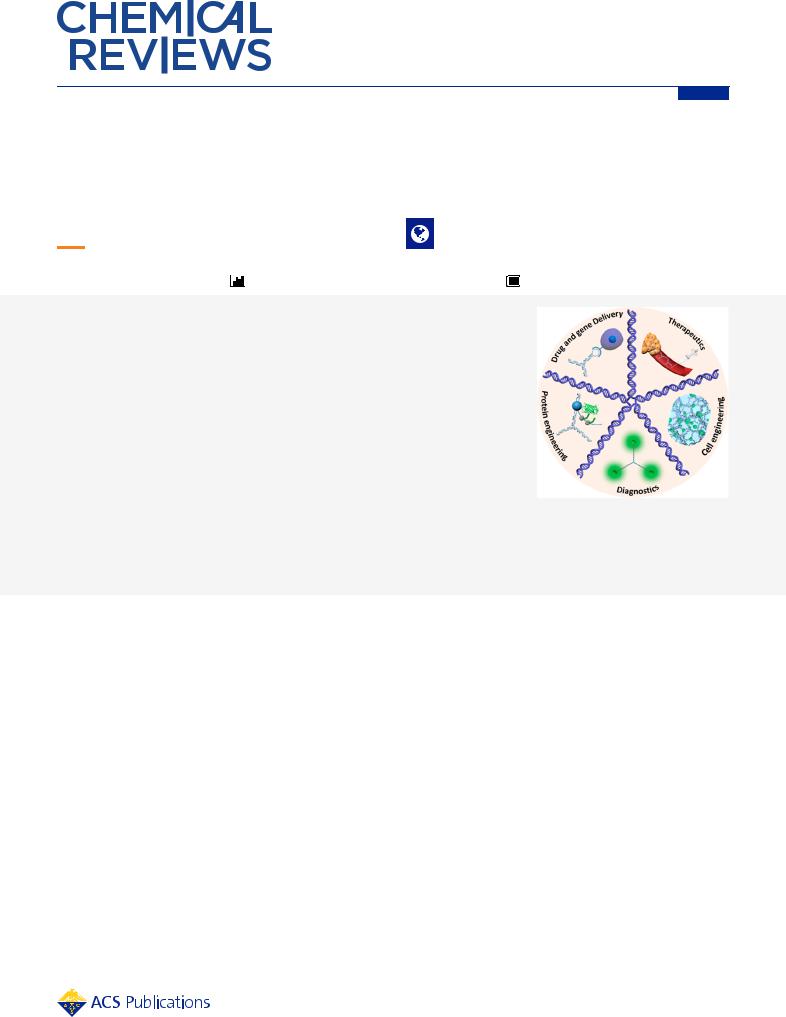
July 16, 2020 at 12:39:15 (UTC). |
on how to legitimately share published articles. |
Downloaded via CARLETON UNIV on |
https://pubs.acs.org/sharingguidelines for options |
|
See |
pubs.acs.org/CR |
Review |
DNA Functional Materials Assembled from Branched DNA: Design, Synthesis, and Applications
Yuhang Dong,§ Chi Yao,§ Yi Zhu, Lu Yang, Dan Luo, and Dayong Yang*
|
Cite This: https://dx.doi.org/10.1021/acs.chemrev.0c00294 |
|
|
Read Online |
|
|||
|
|
|
|
|
|
|
|
|
|
|
|
|
|
|
|
|
|
ACCESS |
|
Metrics & More |
|
|
|
Article Recommendations |
|
|
|
|
|
|
|
||||
|
|
|
|
|
||||
|
|
|
|
|
|
|
|
|
ABSTRACT: DNA is traditionally known as a central genetic biomolecule in living systems. From an alternative perspective, DNA is a versatile molecular building-block for the construction of functional materials, in particular biomaterials, due to its intrinsic biological attributes, molecular recognition capability, sequence programmability, and biocompatibility. The topologies of DNA building-blocks mainly include linear, circular, and branched types. Branched DNA recently has been extensively employed as a versatile building-block to synthesize new biomaterials, and an assortment of promising applications have been explored. In this review, we discuss the progress on DNA functional materials assembled from branched DNA. We first briefly introduce the background information on DNA molecules and sketch the development history of DNA functional materials constructed from branched DNA. In the second part, the synthetic strategies of branched DNA as building-blocks are categorized into base-pairing assembly and chemical bonding. In the third part, construction strategies for the branched DNA-based functional materials are comprehensively summarized including tile-mediated assembly, DNA origami, dynamic
assembly, and hybrid assembly. In the fourth part, applications including diagnostics, protein engineering, drug and gene delivery, therapeutics, and cell engineering are demonstrated. In the end, an insight into the challenges and future perspectives is provided. We envision that branched DNA functional materials can not only enrich the DNA nanotechnology by ingenious design and synthesis but also promote the development of interdisciplinary fields in chemistry, biology, medicine, and engineering, ultimately addressing the growing demands on biological and medical-related applications in the real world.
CONTENTS |
|
4.1. Diagnostics |
AF |
||
1. Introduction |
B |
4.1.1. Sandwich-Based Assay |
AG |
||
4.1.2. Target-Induced Polymerization |
AI |
||||
2. Construction of Branched DNA Building-Blocks |
E |
||||
4.1.3. Target Recycling Strategy |
AJ |
||||
2.1. Base-Pairing |
E |
||||
4.1.4. Multiplexed Diagnostics |
AL |
||||
2.1.1. Static Self-Assembly |
E |
||||
4.2. Protein Engineering |
AM |
||||
2.1.2. Dynamic Self-Assembly |
G |
||||
4.3. Drug and Gene Delivery |
AN |
||||
2.2. Chemical Bonding |
H |
||||
4.3.1. Drug Delivery |
AN |
||||
2.2.1. Transition Metals |
I |
||||
4.3.2. Gene Delivery |
AO |
||||
2.2.2. Disulfide Species |
I |
||||
4.4. Therapeutics |
AP |
||||
2.2.3. Organic Molecules |
I |
||||
4.4.1. Chemo-Gene Therapy |
AP |
||||
2.3. Enzymatic Extension |
J |
||||
4.4.2. Immunotherapy |
AR |
||||
3. Construction of Artificial Branched DNA-Based |
|
||||
|
4.5. Cell Engineering |
AT |
|||
Functional Materials |
K |
||||
5. Conclusion and Outlook |
AT |
||||
3.1. Tile-Mediated Assembly |
K |
||||
Author Information |
AV |
||||
3.2. DNA Origami |
U |
||||
Corresponding Author |
AV |
||||
3.3. Dynamic Assembly |
W |
||||
|
|
|
|||
3.4. Hybrid Assembly |
Z |
|
|
|
|
3.4.1. DNA-Inorganic Nanoparticles Hybrids |
Z |
Received: |
April 13, 2020 |
|
|
3.4.2. DNA-Small Organic Molecules Hybrids |
AB |
|
|||
|
|
|
|||
3.4.3. DNA-Organic Molecule/Transition Metal |
|
|
|
|
|
Hybrids |
AD |
|
|
|
|
3.4.4. DNA-Peptide/Protein Hybrids |
AF |
|
|
|
|
4. Applications |
AF |
|
|
|
© XXXX American Chemical Society |
https://dx.doi.org/10.1021/acs.chemrev.0c00294 |
A |
Chem. Rev. XXXX, XXX, XXX−XXX |
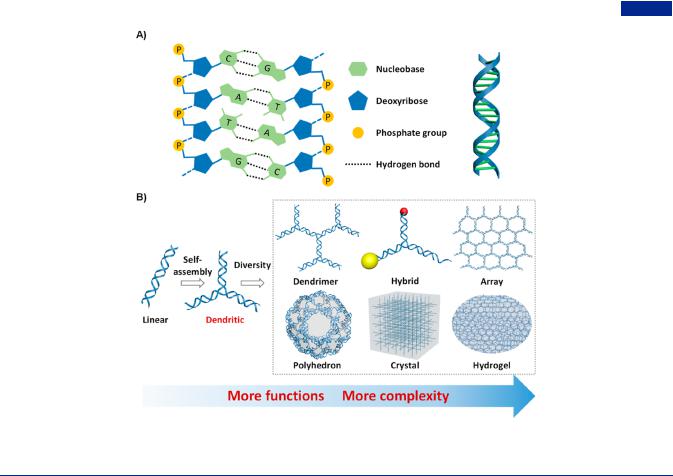
Chemical Reviews |
pubs.acs.org/CR |
Review |
Figure 1. Structures of DNA double helix, branched DNA, and branched DNA-based materials. (A) Double-helix structures of DNA molecules. (B) Branched DNA is assembled from linear DNA, and further assembles into diverse DNA architectures with complex topologies such as dendrimer, hybrid with functional moieties, array, polyhedron, crystal, and hydrogel.
Authors |
AV |
Author Contributions |
AV |
Notes |
AV |
Biographies |
AV |
Acknowledgments |
AW |
Abbreviations |
AW |
References |
AX |
1. INTRODUCTION
Deoxyribonucleic acid (DNA) is traditionally regarded as the genetic molecule in living systems. Inspired by replicational junction in DNA replication and Holliday structure in genetic recombination,1 Seeman et al. in 1982 first proposed the rules on the construction of DNA branched junctions by means of Watson−Crick base-pairing, opening a new era of DNA nanotechnology.2−5 In the following decades, DNA nanotechnology experienced development. DNA is now widely accepted and applied as a generic polymeric material to construct more complex microand nanoarchitectures in the material world.6−12
DNA, as a generic material with sequence programmability, is built up with four di erent deoxynucleotide monomers.4 Each monomer is composed of one of four nitrogen-containing nucleobases (cytosine [C], guanine [G], adenine [A], or thymine [T]), a deoxyribose, and a phosphate group (respectively marked in green, blue and orange color in Figure 1A). The deoxyribose of one monomer and the phosphate group of the next monomer form a phosphodiester bond. The ordered and continuous linkage of a certain number of monomers through phosphodiester bonds results in a deoxynucleotide chain, termed as single-stranded DNA (ssDNA).13 According to
Watson−Crick base-pairing principle, two ssDNA form a double-helix structure, termed as double-stranded DNA (dsDNA). In detail, two hydrogen bonds form between A and T, and three hydrogen bonds form between C and G, resulting in the base pairs with specific complementary sequences as the inner side of double-helix structure.14−16 As a result, two polymeric chains form double-helix structure in antiparallel directions (Figure 1A).
Although DNA molecules were made up of simple units based on established criterion, the diverse deoxynucleotide chains and flexible conformations can be achieved by precise design and user-defined organization. In artificially constructed DNA duplexes, the sequence and number of four nucleobases are arranged precisely as desired, determining the diversity of deoxynucleotide chain. In addition, the flexible conformations of DNA are influenced by sequence-specific base stacking interactions, which are determined by regulating DNA sequences and external environment.17−20 With the development of technologies available to manipulate DNA,21 considerable DNA-based rigid motifs have been prepared to construct di erent dimensional nanostructures as biomolecular sca olds or nanoparticle templates22−26 such as DNA tile assemblies,27,28 DNA origami assemblies,29−32 and DNA dynamic nanomechanical devices.33−38 A variety of DNA-based functional materials have been demonstrated potential for a variety of biological applications, owing to their inherent physiological functions, biocompatibility, and biodegradability.39−42 The development of DNA nanotechnology was promoted by the increasing demand, which in turn facilitated the exploitation of new functional materials, meeting the huge demand in biological and medical-related applications such as tissue engineering and
B |
https://dx.doi.org/10.1021/acs.chemrev.0c00294 |
|
Chem. Rev. XXXX, XXX, XXX−XXX |
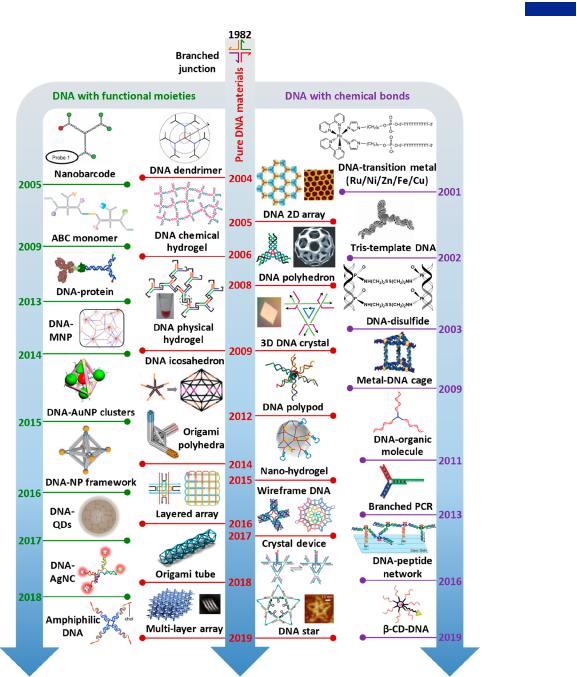
Chemical Reviews |
pubs.acs.org/CR |
Review |
Figure 2. Development history of DNA functional materials constructed from branched DNA. Branched DNA-based functional materials were originated from branched junction and developed into three major categories: pure DNA materials (marked with red), DNA with functional moieties (marked with green), and DNA with chemical bonds (marked with purple). Representative works were displayed alongside the timeline. Pure DNA materials: DNA dendrimer. Adapted with permission from ref 57. Copyright 2004, Springer Nature. DNA 2D array. Adapted with permission from ref 58. Copyright 2005, American Chemical Society. DNA chemical hydrogel. Adapted with permission from ref 59. Copyright 2006, Springer Nature. DNA polyhedron. Adapted with permission from ref 60. Copyright 2008, Springer Nature. DNA physical hydrogel. Adapted with permission from ref 61. Copyright 2009, Wiley-VCH. 3D DNA crystal. Adapted with permission from ref 62. Copyright 2009, Springer Nature. DNA icosahedron. Adapted with permission from ref 63. Copyright 2009, Wiley-VCH. DNA polypod. Adapted with permission from ref 64. Copyright 2012, American Chemical Society. Origami polyhedral. Adapted with permission from ref 65. Copyright 2014, American Association for the Advancement of Science. Nanohydrogel. Adapted with permission from ref 66. Copyright 2015, American Chemical Society. Wireframe DNA. Adapted with permission from ref 67. Copyright 2015, Springer Nature. Layered array. Adapted with permission from ref 68. Copyright 2016, Wiley-VCH. Crystal device. Adapted with permission from ref 69. Copyright 2017, Springer Nature. Origami tube. Adapted with permission from ref 70. Copyright 2018, American Chemical Society. DNA star. Adapted with permission from ref 71. Copyright 2019, Springer Nature. Multilayer array.72 DNA with functional moieties: Nanobarcode. Adapted with permission from ref 73. Copyright 2005, Springer Nature. ABC monomer. Adapted with permission from ref 74. Copyright 2009, Springer Nature. DNA−protein. Adapted with permission from ref 75. Copyright 2013, American Chemical Society. DNA-MNP. Adapted with permission from ref 76. Copyright 2017, American Chemical Society. DNA-AuNP clusters. Adapted with permission from ref 77. Copyright 2015, American Chemical Society. DNA-NP framework. Adapted with permission from ref 78. Copyright 2016, Springer Nature. DNAQDs.79 DNA-AgNC. Adapted with permission from ref 80. Copyright 2018, Wiley-VCH. Amphiphilic DNA. Adapted with permission from ref 81. Copyright 2018, American Chemical Society. DNA with chemical bonds: DNA-transition metal (Ru/Ni/Zn/Fe/Cu). Adapted with permission from ref 82. Copyright 2001, Wiley-VCH. Tris-template DNA. Adapted with permission from ref 83. Copyright 2002, Springer Nature. DNA-disulfides.
C |
https://dx.doi.org/10.1021/acs.chemrev.0c00294 |
|
Chem. Rev. XXXX, XXX, XXX−XXX |

Chemical Reviews |
pubs.acs.org/CR |
Review |
Figure 2. continued
Adapted with permission from ref 84. Copyright 2003, American Chemical Society. Metal-DNA cage. Adapted with permission from ref 85. Copyright 2009, Springer Nature. DNA-organic molecule. Adapted with permission from ref 86. Copyright 2011, American Chemical Society. Branched PCR. Adapted with permission from ref 87. Copyright 2013, Wiley-VCH. DNA-peptide network. Adapted with permission from ref 88. Copyright 2016, Wiley-VCH. β-CD-DNA. Adapted with permission from ref 89. Copyright 2019, American Chemical Society.
Figure 3. Overall framework of the review including types of branched DNA building blocks in Chapter 2 and construction of branched DNA-based materials in Chapter 3. The types of branched DNA building-block are branched junction, three-point-star motif, DNA tensegrity triangle, and branched DNA with chemical bonds, abbreviated as B, S, T, and C in the blue circle, respectively. The construction strategy of branched DNA-based materials is classified as tile-mediated assembly, DNA origami, dynamic assembly, and hybrid assembly, abbreviated as T, O, D, and H in the orange square respectively, followed by the conceptual assembly procedure as classification. The representative functional materials are constructed from branched DNA including dendrimer, polyhedron, array, crystal, hydrogel, and hybrids. The building-blocks and construction strategies in the reported work are listed under the corresponding materials.
therapeutics. DNA nanotechnology gradually became a booming field in materials science and engineering.
In general, as versatile molecular building-blocks to construct functional materials, the topologies of artificial DNA polymeric chains include linear, circular, and branched types.43 The linear and circular DNA are most common naturally existing forms of DNA. The linear DNA is the easiest form to design and synthesize, thus it is commonly utilized as the basic unit for organizing various nanomaterials.44 The circular DNA can act as sca olds for the construction of DNA architectures such as rotaxane-like DNA structures45,46 and DNA nanotubes.47 However, there are still certain limitations in using circular DNA as building-blocks. On the one hand, owing to the occurrence of bending and twisting sti ness, dsDNA is a
relatively rigid polymer, which has a persistence length of 150 bp (about 50 nm) and an optimal cyclization probability of 500 bp,48−50 making di cult to form circular dsDNA with small size only by base stacking force. On the other hand, to form small circular DNA, some organic molecules or enzymes need to be introduced.51,52 The introduction of additional components will increase the complexity of operation. In addition, the closed ring structure makes circular DNA impossible to self-expand.
Remarkably, to be beneficial for DNA structures spanning from multiple dimensions, branched structure needs introducing. Branched DNA possesses more complex topologies and functional modules and thus can overcome the limitations of structures and applications of linear DNA and circular DNA.53 In terms of topology, the classical branched DNA can be
D |
https://dx.doi.org/10.1021/acs.chemrev.0c00294 |
|
Chem. Rev. XXXX, XXX, XXX−XXX |

Chemical Reviews |
pubs.acs.org/CR |
Review |
assembled form linear DNA, which is composed of multiarm dsDNA or ssDNA elongating from a branched point (Figure 1B).3,54 With rational design, the branched DNA monomers can generate well-defined and high-ordered two-dimensional (2D) or three-dimensional (3D) DNA nanostructures through the self-assembly of sticky ends, such as dendrimer, array, polyhedron, crystal, and hydrogel (Figure 1B).55,56 In terms of functions and applications, the multivalent branches endow branched DNA the capability to conjugate with di erent functional components, called DNA hybrids, which enable a variety of applications in the fields of biomaterial and biomedicine.53
In principle, branched DNA conforms to the definition of dendrimers: a hyper-branched polymer originates from a central core and then grows into a highly controlled and well-defined macromolecule by an iterative reaction.90−92 However, unlike conventional chemical dendrimers with isotropic structures,93 branched DNA can be designed to be either isotropic or anisotropic, and be symmetrical or asymmetrical, which makes branched DNA a flexible, versatile, and ideal building-block.94 In general, the characteristics of branched DNA include the following: (i) they can act as either initiator cores or growing building-blocks for the construction of hyper-branched DNA nanostructures in a controlled fashion; (ii) the sequences on branched DNA can be rationally designed according to the desired requirements; and (iii) the customized sticky-ends on branched DNA provide multiple modified sites to connect with specific functional moieties.43,95 Consequently, branched DNA not only possesses the properties of general DNA materials, such as programmability, manipulability, precise structure, molecular recognition ability, and biological function, but also have the advantages of size-tunability, multivalency, and controllable symmetry.96,97 In fact, few other materials possess all of the aforementioned advantages as branched DNA does; thus, branched DNA has gradually become an attractive, versatile, and promising building-block for the construction of highly complicated architectures.39,98 As shown in Figure 2, the timeline illustrates the development history of branched DNAbased materials. Branched junction as the primary nanostructure unit derived three major forms of branched DNA-based materials including pure DNA materials (marked with red), DNA with functional moieties (marked with green), and DNA with chemical bonds (marked with purple). The branched DNA-based pure materials exhibited the development trend of “more functions, more complexity”, from static structures (i.e., 3D DNA crystal) to dynamic structures (i.e., crystal device), from plane structure (i.e., 2D array) to steric structure (i.e., DNA polyhedron), from irregular shape (i.e., DNA dendrimer) to highly ordered array (i.e., crystal), from micro (i.e., DNA polypod) to macro (i.e., hydrogel), from single-layer to multilayer. On the basis of pure branched DNA nanostructures, many functional moieties were introduced to construct functional branched DNA-based nanostructures such as fluorescent groups, photo-cross-linkable groups, hydrophobic groups, inorganic nanoparticles, and biomolecules. In addition, chemical covalent bonds could be introduced into branched DNA structures in replace of hydrogen bonds to form branched DNA with enhanced stability. In the past decades, branched DNA has undergone a sustainable development from control of precise structure to multifunctions. Recently, a variety of engineered branched DNA-based functional materials have been created, and many promising applications have been explored.
In this review, we are focusing on the recent research progress on the engineering of branched DNA-based functional materials (Figure 3). First, we introduce two major strategies for the construction of four types of branched DNA building-blocks including base-pairing and chemical bonding. Second, we summarize the construction of branched DNA-based materials including tile-mediated assembly, DNA origami, dynamic assembly, and hybrid assembly. Third, applications particularly in biological-related fields are demonstrated including diagnostics, protein engineering, drug and gene delivery, therapeutics, and cell engineering. In the end, we provide an insight into the remaining challenges and future perspectives toward branched DNA-based functional materials in biological applications.
2.CONSTRUCTION OF BRANCHED DNA BUILDING-BLOCKS
Since Seeman et al. proposed the rules on the construction of DNA branched junctions, many self-assemblies with geometric rigidity and high-controlled topologies have been fabricated,99−105 which broke the restriction of using linear duplex DNA as building-blocks. Branched DNA monomer as a building-block was traditionally composed of multiple-armed DNA strands derived from branched points.106−108 The branched point of branched DNA could be either a nucleoside or a non-nucleoside unit. Depending on the types of branched points, the construction methods of branched DNA buildingblocks are mainly categorized into base-pairing and chemical bonding.
2.1. Base-Pairing
The base-pairing rule indicated the specific recognition of A-T and C-G, which was the most predominant approach by virtue of hydrogen bonds and base-stacking as noncovalent force.14 Base- pairing-based methods were simple and convenient to manipulate, mainly including static self-assembly and dynamic self-assembly.
2.1.1. Static Self-Assembly. Branched DNA monomers are easily accessible through the static self-assembly of constituent oligonucleotide strands with partially complementary to each other.69,109 Each arm usually contains a double-stranded region and an extended sticky end. The crossed double-stranded regions are used to tighten complementary ssDNA to maintain the rigidity and stability of the structures. In addition, the flexible sticky ends could elongate branched DNA into DNA networks or connect multiple functional elements.110,111 As a result, branched DNA could act as building-blocks for the assembly of complex DNA superstructures that possess well-defined structures and additional functionalities.112−114
In general, the key to the successful synthesis of branched DNA based on static base-pairing is the rational design of oligonucleotide sequences. The design principles mainly include
(1) a high binding energy of base pairs is necessary to overcome the energy barrier. Usually, the binding energy is closely associated with the Gibbs free energy ( G) and melting temperature (Tm) of double-helix region, which is optimized by regulating the base composition and length of ssDNA; (2) the formation of stable secondary structures of ssDNA should be avoided; (3) the oligonucleotide sequences should be asymmetric to avoid the migration of branch point. In addition, owing to the strong electronegativity of DNA, cations with appropriate species and concentration are required to overcome the electrostatic repulsion of ssDNA, thus ensuring the stability
E |
https://dx.doi.org/10.1021/acs.chemrev.0c00294 |
|
Chem. Rev. XXXX, XXX, XXX−XXX |
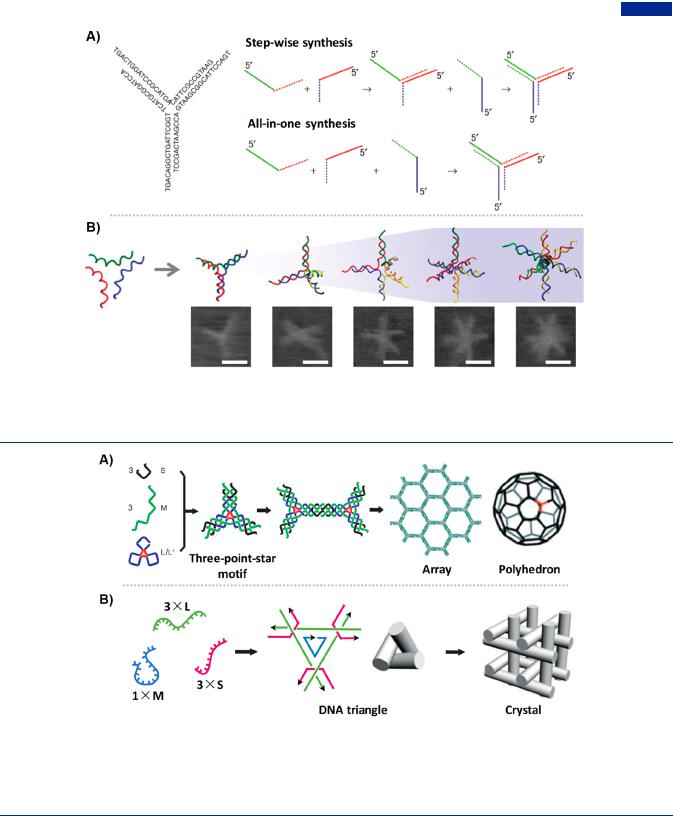
Chemical Reviews |
pubs.acs.org/CR |
Review |
Figure 4. Synthetic methods of branched DNA based on base-pairing. (A) Y-shaped branched DNA prepared by two synthetic methods: stepwise synthesis and all-in-one synthesis. Adapted with permission from ref 57. Copyright 2004, Springer Nature. (B) Multibranched DNA nanostructures self-assembled from multiple oligonucleotide chains with partially complementary sequences. Scale bars represented 20 nm. Adapted with permission from ref 64. Copyright 2012, American Chemical Society.
Figure 5. Construction of three-point-star branched DNA and DNA tensegrity triangle based on base-pairing. (A) Fabrication method of three-point- star branched DNA and derived high-ordered structures. Three-point-star branched DNA was self-assembled from seven individual single-strands, including a long repetitive strand, three fixed strands, and three short strands. On the right were various high-ordered structures derived from three- point-star branched DNA including DNA array58 and DNA polyhedron.60 Adapted with permission from ref 60. Copyright 2008, Springer Nature. (B) Fabrication of DNA tensegrity triangle from L, M, and S strands at a 3:1:3 stoichiometric ratio. The DNA triangle as a rigid motif was used to produce 3D crystalline lattice on macroscale. Adapted with permission from ref 129. Copyright 2015, Wiley-VCH.
of branched DNA.115,116 After the reasonable design of sequences, branched DNA is self-assembled by simple mixing of corresponding ssDNA and optimized annealing procedures, where hydrogen bonds are formed autonomously between complementary base pairs.
One of the topological structures of branched DNA monomers was single double-helix as an arm of branches. In 2004, Li et al. first synthesized Y-shaped branched DNA (Y- DNA) as repetitive units of dendrimer-like DNA (DL-DNA).57
The Y-DNA with single double-helix as an arm was selfassembled from three ssDNA that were partially complementary to each other (Figure 4A). They introduced two strategies for the preparation of monodisperse Y-shaped DNA including stepwise synthesis and all-in-one synthesis; the yield of Y-DNA reached nearly 100% by both strategies. Y-DNA remained intact at 4 °C without degradation in 30 days. The configuration and flexibility of Y-DNA was regulated by the length of each arm and external temperature.117 Y-shaped DNA in solution showed a
F |
https://dx.doi.org/10.1021/acs.chemrev.0c00294 |
|
Chem. Rev. XXXX, XXX, XXX−XXX |
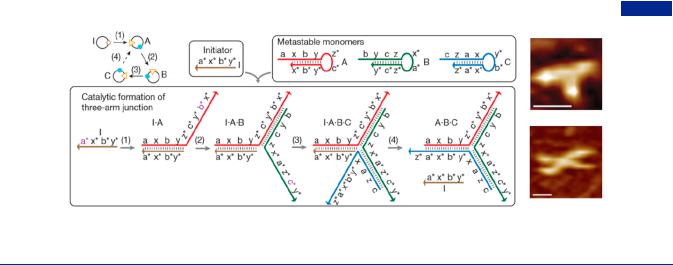
Chemical Reviews |
pubs.acs.org/CR |
Review |
Figure 6. Dynamic assembly and disassembly pathways of branched DNA based on CHA reaction including an initiator and metastable hairpin monomers. On the right were atomic force microscope (AFM) images of a three-branched DNA and a four-branched DNA, respectively. Scale bar, 10 nm. Adapted with permission from ref 130. Copyright 2008, Springer Nature.
nonplanar pyramid structure and a hollow cavity appearing at branched center, where adjacent complementary bases were unpaired.118 This is mainly due to the steric strain and base stacking interactions occupying the prominent position rather than hydrogen bonding. In addition to Y-DNA, multibranched DNA structures surrounding a branched point have been prepared as such topology with single double-helix as an arm by this synthetic method (Figure 4B).64,115,119 The di erent number (also termed as valence) and length of branches could a ect equilibrium phase behaviors of branched DNA, including phase critical temperature and concentrations, showing the behaviors of colloidal particles.120−122
Although fabricating branched DNA with single double-helix as an arm was simple to design and easy to implement, a limitation existed for this fabrication method: the synthetic di culty increased over the branch number of branched DNA due to the inherent molecular tension and electrostatic repulsion of DNA. To overcome this limitation, Mao and co-workers developed a novel fabrication method for building another topology of branched DNA monomers with multiple doublehelix as an arm in 2008 (Figure 5A).60 They introduced a central loop into branched DNA to relieve molecular tension. In detail, they constructed symmetric three-point-star branched DNA as identical elements. The three-point-star branched DNA with rotational symmetries was composed by three parts: a long repetitive strand with single-strand loop as core (marked with blue and red), three fixed strands (marked with green), and three short strands as periphery for elongation (marked with black). Each arm of three-point-star branched DNA contained two double-helix structures extended from a central loop. The existence of loop avoided the molecular stress between flank branches. The number and length of loop influenced the number of identical branches and the flexibility of elements, respectively.58 In addition, the turnover of DNA duplex on arm was used to control the curvatures of the formed structures via corrugation strategy, whether planar or nonplanar.123,124 Through rationally designing the length of loop and the turnover of DNA duplex, the three-point-star branched DNA hierarchically self-assembled to form high-ordered hexagonal 2D arrays
and a series of 3D DNA polyhedrons via base-pairing in one-pot synthesis (Figure 5A).58,60,125−128
To meet the requirements of more stability of DNA architectures, a new conformation of branched DNA monomer called DNA tensegrity triangle was developed (Figure 5B). DNA tensegrity triangle was fabricated from three types of strands, L, M, and S strands at a defined stoichiometric ratio, where L and
M strands assembled into triangle framework and S strands with cohesive ends was responsible for the extension of motifs. Three helices were connected in a crossover form to produce six extension directions that were not on a plane. The yielded DNA tensegrity triangle was presented as 3-fold rotational symmetry. In the perspective of mechanical property, the relatively rigid double-helix region enforced the mechanical strength of the structure, and relatively flexible ssDNA allowed the structure to maintain three internal angles at 60 °C at a relaxed state. By virtue of tensegrity equilibrium between sti multihelix branches and relaxed sticky ends, DNA tensegrity triangle could selfassembled macroscopic 3D crystals with rhombohedral lattice and a desired di raction resolution by adjusting the edge length. The kind of branched DNA with tensegrity structure was extensively used in the construction of well-defined 3D crystals.
2.1.2. Dynamic Self-Assembly. With the development of DNA dynamic structural chemistry, a novel method called catalyzed hairpin assembly (CHA) has attracted increasing attentions for dynamic self-assembly of branched DNA.130−132 As shown in Figure 6, a single-stranded initiator and several metastable hairpin monomers were involved in the assembly and disassembly pathways. These initiators and hairpin monomers possessed specified sequence and precise structure to meet the requirements of dynamic reaction. In a CHA reaction, the initiator was complementary to an exposed toehold of a hairpin chain, and further opened the hairpin structure driven by sequence-dependent free energy of base pairs, which was called toehold binding and branch migration, respectively.34 The opened hairpin DNA exposed a new single-stranded region, which in turn served as the triggering strand for opening another hairpin chain. As a result, branched DNA was formed by the cascaded opening of metastable hairpin chains triggered by initiator, namely assembly pathway. When the last hairpin chain replaced the released the initiator, the disassembly pathway took place. The initiators in the CHA reaction played two roles: catalysts to reduce the activation energy and specifically trigger the subsequent reaction; recyclable reagents to be released and used for the next round of CHA reaction. In the absence of initiators, the hairpins with storage energy in loop failed to undergo the conformational transition due to the higher energy barrier and kinetic trap.133,134 In addition, other than traditional annealing method with tedious control over the temperature, the isothermal CHA occurred only depending on the principle of base-pairing. The characteristics of CHA foresee promising applications in the fields of isothermal nucleic acid amplification,
G |
https://dx.doi.org/10.1021/acs.chemrev.0c00294 |
|
Chem. Rev. XXXX, XXX, XXX−XXX |
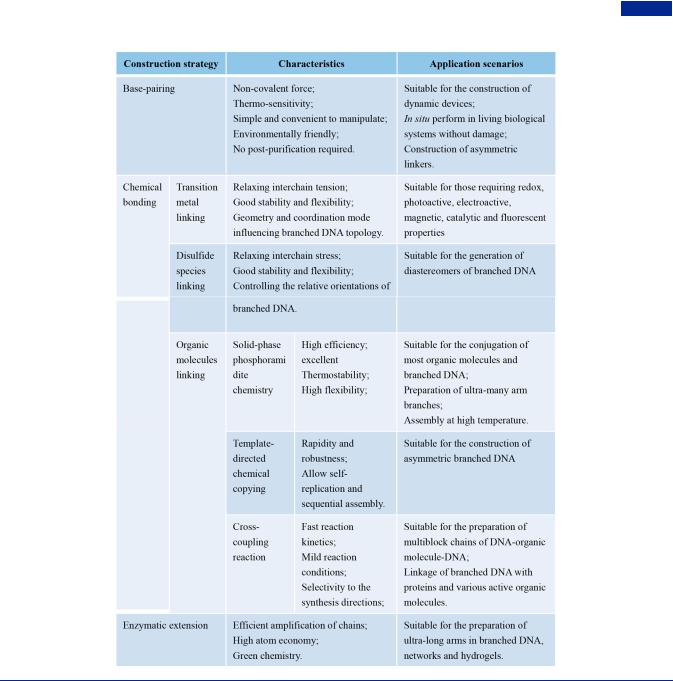
Chemical Reviews |
pubs.acs.org/CR |
Review |
Table 1. Summary of Construction Methods for Branched DNA Building-Blocks
biomolecular detection, molecular machine, and comput-
ing.135−138
The cascaded deformation of hairpin DNA allowed CHA reactions to be applied in the construction of concatenated logic circuit.139 In a concatenated logic circuit, three individual hairpin chains were used as inputs and outputs simultaneously. The design of CHA reaction made it possible that the security of logic circuit depended on not only the right combination, but also the proper order of hairpin chains as inputs. In addition, the logic circuit can be reset and recycled to the initial state without external stimuli owing to the spontaneous release of initiators. Finally, the logic circuits output the signals by color changes, which contributed to the implant of G-quadruplex DNAzyme with peroxidase-like activity. The logic circuits constructed from CHA reactions therefore possessed the features of biocomputing security, automatic reset, and naked-eye recognition.
2.2. Chemical Bonding
Chemical modifications of 5′ or 3′ ends on DNA provided tremendous potentials for the conjugation with other chemicals.6,140,141 Chemical bonding is typically amenable to the linkage of DNA with various non-nucleoside groups, enriching the formation of branched DNA. These substrates provided tethered cores and customized positions for the ordered aggregations of oligonucleotide chains, introducing extra structural or chemical properties to branched DNA.142,143 In addition, the incorporation of chemical bonding e ectively relieved the interchain tension in branched DNA, and improved the flexibility and thermostability. A variety of synthetic nonnucleic acid molecules could serve as branch points for constructing branched DNA such as metal-centered complexes, disulfide species, and organic molecules.144−146 The constructions methods for branched DNA monomers were summarized in Table 1 including the characteristics and applicable scenarios.
H |
https://dx.doi.org/10.1021/acs.chemrev.0c00294 |
|
Chem. Rev. XXXX, XXX, XXX−XXX |

Chemical Reviews |
pubs.acs.org/CR |
Review |
Figure 7. Chemical bonds as branched cores of branched DNA. (A) Branched DNA structure with luminescent transition metal (Ru)-centered molecules as branch points. Adapted with permission from ref 82. Copyright 2001, Wiley-VCH. (B) Branched DNA-disulfide cross-linked structure. Adapted with permission from ref 84. Copyright 2003, American Chemical Society. (C) Tribranched organic molecule-dsDNA nanostructure. Adapted with permission from ref 86. Copyright 2011, American Chemical Society.
2.2.1. Transition Metals. Some transition metals have been demonstrated as the branch points of branched DNA structures.147 In 2001, Sleiman group first synthesized a class of metal-branched DNA complexes with regular geometric shape via the solid-phase synthesis. The metal-branched DNA complexes contained two parallel DNA strands and transition metal as the branch point (Figure 7A).82 The geometries and coordination mode of transition metal center directly influenced the arrangement of oligonucleotide chains and arm numbers of branched DNA. They introduced cis-[(bpy)2Ru(imidazole)2]2+ as transition metal into branched DNA, which possessed redoxand photoactive, and enhanced fluorescent properties. To control the flexibility of metal-centered DNA complex, they applied Ru(II) tris(bipyridine) molecules with relative rigidness as metal centers. When two parallel DNA chains had complementary sequences, Ru(II)-centered DNA complexes would bring together to self-assemble into a discrete cyclic metal-DNA complex.148 Further, two Ru(II)-centered DNA with complementary sequences as symmetrical building-blocks attributed to the construction of a library of assemblies including predicted oligomers, DNA ladders, and microsized DNA fibers.149 In addition to Ru(II)-centered multiarm DNA complex, Ni(II)-cyclam-centered, Fe(II)-centered, and Zn(II)- porphyrin-centered multiarm DNA conjugates were prepared as precursors for the generation of branched DNA and supramolecular DNA assemblies.150−154 Sleiman and co-workers further developed a template strategy for the incorporation of metal ions (Cu(I), Cu(II), and Ag(I)) as branch points into branched DNA.155 They took advantage of the coordination bonds of metal ions and ligand (diphenylphenanthroline, dpp) modified on DNA to prepare three-branched DNA-metal(dpp)2 complexes, which had the characteristic of electroactivity, chirality transfer, and enhanced stability. In addition, they introduced specific DNA to realize the dynamic assembly of branched DNA-metal nanostructures. The transition-metal
coordinated DNA complexes showed great potential in building 3D DNA objects such as DNA nanotubes and DNA prism.85,156
Duprey et al. proposed metal-mediated base-pairing to construct three-arm branched DNA interlocked by a ligand (bpy)-metal
(Ni) complexation (3:1) as branching point.157 In general, these transition metal-ligand compounds as branch points could influence the orientation and conformation of DNA strands, and increase the stability of branched DNA by metal-ligand coordination in replace of natural hydrogen bonds.157 Moreover, the flexible coordination bonds enabled the construction of dynamic metal-DNA assemblies.155,158 The incorporation of transition metal into branched DNA endowed the complexes with addressable configuration, enhanced stability, intense fluorescence, and redox activity.4
2.2.2.Disulfide Species. Disulfide species, behaving as stable and covalent linkages, were employed as one of common groups to control the relative orientations of branched DNA. In 2003, Endo et al. assembled branched DNA structures (XLDNA) by a cross-linker containing disulfide linkage, in which
two oligonucleotide strands were in a relatively controlled position (Figure 7B).84 The disulfide linkage was modified on the phosphorus atoms outside the DNA chain to relax molecular stress of XL-DNA. Two XL-DNA and complementary strands could self-assemble into multibranched DNA nanostructures in one-pot synthesis. In addition, because the disulfide linkage was modified in an opposite direction to generate two diastereomers,
the relative arrangement of multibranched DNA was controlled in parallel or antiparallel orientations.159 The stereoisomerism of
XL-DNA a ected the flexibility of disulfide linkage and the stability of multibranched DNA.144,145
2.2.3.Organic Molecules. Organic molecules were used as
the building-block cores of branched DNA, whose active groups reacted with modified ssDNA to form branching chain.160,161 The methods for coupling branched DNA with organic molecules were generally divided into three categories, namely solid-phase phosphoramidite chemistry, template-directed chemical copying, and cross-coupling reactions.
Traditional solid-phase phosphoramidite chemistry was one of the earliest methods applied in the synthesis of branched DNA with organic molecules as building-block cores.162,163 The arm number of branched DNA primarily relied on the reagents branches tethered on the surface of a solid glass support; for instance, a trebling reagent (also called synthon) as a branch
I |
https://dx.doi.org/10.1021/acs.chemrev.0c00294 |
|
Chem. Rev. XXXX, XXX, XXX−XXX |

Chemical Reviews |
pubs.acs.org/CR |
Review |
core extended to form trioligonucleotides in a standard solidphase phosphoramidite protocol.160 The resulting branched synthon was coupled to synthesize multigeneration of branched DNA with organic molecules as cores. The assembled duplex had higher thermostability than linear counterparts judged from melting temperature.162,164 More than that, the branched DNA hybrid with adamantane as an organic core even formed a new material when reaching 95 °C in case of only two palindrome complementary bases.165 This adamantane-core branched DNA could be assembled with eight arms simultaneously, almost reaching the limit of arm number, and could derivate into DNAprotein hybrids under the manipulation of ligase enzymes.161,166 In addition, the geometry and flexibility of organic-molecule cores and the length of spacer units both a ected the assembly of branched DNA in well-defined supramolecular conformations, which were demonstrated by synergistic experimental study and molecular dynamics simulations.167,168 The highly flexible cores (e.g., tris(oxypropyloxymethyl)methyl) tended to form cage dimers, while relatively rigid organic cores (e.g., tetraphenylmethane) greatly biases the assembly of polymeric nanoparticles.169 The nanoparticle assemblies had the narrowly dispersed size and size-tunability by controlled-adjusting assembly conditions, including monomers concentrations, time, and salt concentrations, as well as good cellular internalization and DNase resistance.
On the basis of phosphoramidite chemistry, a novel strategy
called DNA template-directed chemistry reactions was proposed.140,170 Eckardt et al. first proposed the strategy of
template-directed chemical copying for the synthesis of threearm branched DNA extended from a tris-organic backbone.83 Three individual hydrazide-modified ssDNA reacted with a trislinker through hydrazine-formation reaction and generated branched DNA nanostructures. The presence of tris-oligonu- cleotides with complementary sequences as template improved the yield of expected branched DNA structures owing to the specific recognition of base-pairing. By taking advantage of template-directed chemical copying, Zimmermann et al. generated asymmetric tris-oligonucleotides from a tris-linker amide for the formation of DNA polyhedron.171
The above-mentioned methods of phosphoramidite chemistry and DNA-templated chemical copying have unavoidable drawback that there existed unchangeable directionality between DNA and organic molecules, which limited the extension of DNA sequences toward both directions. Crosscoupling reactions as one of common methods was flourishing for the synthesis of branched DNA-organic molecule hybrids because of its selectivity to the synthesis directions.86 Moreover, the cross-coupling reactions provided higher yields and product purity, meeting the requirement of subsequent amplification.172 In general, the cross-coupling reactions used for the synthesis of branched DNA with organic molecules as the cores were divided into amide-coupling reaction, isothiourea bond formation, thiolcoupling reaction, and click chemistry. The amide-coupling reaction and isothiourea bond formation reaction typically occur between amino-modified DNA strands and organic molecules containing NHS succinimidyl ester and isothiocyanate to form stable covalent bonds, respectively.172−174 Thiol-coupling reaction usually referred to the covalent linkage between thiol group-conjugated DNA and maleimide molecules.175 Endo et al. synthesized a four-arm DNA-porphyrin unit by coupling tetramaleimide-linked tetraphenylporphyrin and thiol-modified ssDNA, which could self-assemble into high-ordered 2D DNA array and DNA tube.176 Click reactions, also known as Cu-
catalyzed azide−alkyne cycloaddition, as the most common method of cross-coupling reactions had a variety of characteristics of mild reaction conditions, high e ciency, high chemical selectivity, and fast reaction kinetics.177−179 Lee et al. used these coupling methods to build a series of linear and branched DNAorganic molecules (Figure 7C) and observed the highest yield of amide-coupling reaction.86 The thermostability of obtained molecules enabled to construct microsized supramolecular assemblies under high temperature. Lundberg et al. introduced site-specific click chemistry to construct three-branched DNAbenzene units for the addressable assembly of DNA polyhedral nanonetwork.180 They analyzed that the yield of click chemistry followed the binomial distribution and the total yield was a ected by both step-yield and hybridization steps. Paredes et al. utilized the click chemistry and periodic helicity of DNA to tune the dihedral angular between branches in backbone-branched DNA, which facilitated the formation of well-defined DNA nanostructures.181 Gothelf et al. developed a modular programmed assembly with linear and tripoidal DNA-organic molecules as building units.143 The bottom-up modular assembly was amenable to the ordered arrangement of modules into linear or branched extension by metal-Salen coupling reaction and base pairing in accordance with the predetermined action.182,183 These metal-linking branched DNA had the potential as conducers for electronics.184
In general, the backbones of organic molecules o ered predefined and controlled sites for the assembly of branched DNA. In addition, these covalent linkages between organic molecules and modified ssDNA enhanced the flexibility and stability of these DNA-organic hybrid structures, enabling the formation of more complex architectures, such as polyhedron and network.171,180 Moreover, some organic molecules possessing specific characteristics provided additional functions for branched DNA hybrids175 such as photoresponsiveness.185
2.3. Enzymatic Extension
The employment of branched DNA with ultralong strands was indispensable for the preparation of a myriad of functional DNA-based materials and relevant application scenarios, such as DNA hydrogel with gene fragments enabling protein production. However, the arm length of branched DNA synthesized by base pairing or chemistry bonding was limited by the synthetic capability of solid-phase synthesis. Therefore, the method of enzymatic extension came into being, especially polymerase chain reaction (PCR) that was able to amplify DNA fragments e ciently, gradually being a promising and powerful tool in modern biotechnology. Traditional PCR was performed with template DNA and linear DNA primers, and branched PCR enabled the formation of branched DNA products with large fragments by using branched DNA as primers.186 The hydrogen bonds between base pairs (A-T and C-G) were easily destroyed at high temperature, which restricted the applications of branched DNA nanostructures in PCR. To address this problem, some organic molecules were introduced to covalently link branched DNA for the construction of thermostable DNA nanostructures.187−189 Marx groups proposed a method of using covalently cross-linked branched DNA as primers, and constructed 3D DNA network through branched PCR amplification.110,190,191 The branched primers with organic molecules as cores were formed by standard solid phase synthesis, whose length influenced the meshes of DNA network. The method provided a strategy for the addition of chemically modified nucleotides in PCR. Liu et al. used flexible branched
J |
https://dx.doi.org/10.1021/acs.chemrev.0c00294 |
|
Chem. Rev. XXXX, XXX, XXX−XXX |