
диафрагмированные волноводные фильтры / 9fd7b4eb-038a-44bb-af8a-eb4491610e58
.pdf
140GHz Waveguide H ladder Bandpass Filter
Wang Cheng, Lu Bin, Liu Jie, Deng Xianjin
Terahertz Research Center, Institute of Electronic Engineering, China Academy of Engineering Physics 919-523 mail box
,Mianyang, Sichuan, 621900, China
Abstract-A low insertion loss 140GHz rectangular waveguide H ladder bandpass filter is presented in this paper. The filter is synthesized by 7-pole Chebyshev filtering function and simulated by mode matching method. It is fabricated based on micrometer precision high speed CNC milling machines. The measured center frequency is 139.2GHz. The 3dB bandwidth is 11.6GHz, 8.3%. The minimum insertion loss is -1.0dB. The passband return loss is lower than -16.4dB. The stopband rejection is - 48.1dB at 130GHz.
I.INTRODUCTION
Microwave and millimeter wave filters are the critical passive components in wireless communication and radar transceivers based on superheterdyne structure. They are used in wonder frequency band selection, transmitter image signal rejection and receiver image noise rejection. As the developing of high data rate communication over 10Gbit/s and high resolution imaging radar between 1~10cm, it is needed to increase transceiver operation frequency to millimeter and terahertz band, increase transceiver operation bandwidth over 10GHz. Between 2010-2011, Institute of Electronic Engineering (IEE), China Academy of Engineering Physics(CAEP) has developed a kind of 140GHz wireless communication systems [1], which has realized 10Gbps non real time and 2Gbps real time data transmission over 1.5km. A 140GHz bandpass filter developed for 140GHz wireless front end will be presented in this paper.
As the operation frequency increased to millimeter and terahertz wave band, metal loss and surface roughness will greatly increase the filter insertion loss, frequency resonator and coupling structure need micrometer physical precision to maintain useable filter performance, complicated structure is hard to be applied. Frequency Selected Surface (FSS) filter is a candidate of terahertz filter, which exhibits insertion loss <0.3dB at 700GHz under experimental measurement [2] [3]. It has the disadvantages of unsatisfied stopband rejection, hard to integrated in waveguide module, sensitive to installation and environment, fragile. Yuan Li et al. has used deep reactive ion etching technology with stacked silicon substrate to develop a W band waveguide H ladder filter [4], with center frequency 92.45GHz, insertion loss between 1.1dB and 1.3dB, bandwidth 4.83%, 30dB rejection at 9GHz away from center. S. V. Robertson et al. has developed 95GHz filter based on silicon micromachining microstrip [5], bandwidth 12.5%, insertion loss 2.2dB, 30dB rejection at 85GHz.
The development of micrometer precision high speed CNC milling machines make it possible to fabricate filter based on
classical EM theory. We developed a 140GHz filter based on 7-pole waveguide H ladder structure. It has the advantage of low loss, high stopband rejection and low passband return loss. It was designed by mode matching method, the simulation and measured data coincided well with each other.
II.DESIGN AND SIMULATION
A.Filter electromagnetic structure
Figure 1. 140GHz 7-pole waveguide H ladder bandpass filter
The structure of 140GHz waveguide H ladder filter is showed in figure 1. Filter is based on WR06 standard rectangular waveguide (a=1.651mm, b=0.826mm). The filter consists of 7 half wavelength resonator and 8 coupling structure. Detail structure of cascaded section is showed in figure 2. The coupler thickness b1 is all set to 0.5mm to enhance the mechanical strength. Minimum R=0.15mm fillet is added to the edge of coupler to adapt the requirement of high speed CNC milling fabrication.
a |
Resonator |
Resonator |
a1 |
Resonator |
|
Lm-1 |
Lm |
Lm+1 |
|||
|
|
||||
|
|
Side view |
Coupler |
|
|
|
|
|
|
||
|
|
|
b1 |
R |
|
b Resonator |
Resonator |
Resonator |
|||
|
|||||
|
Lm-1 |
Lm |
Coupler |
Lm+1 |
|
|
|
|
|
Top view
Figure 2. Waveguide half wavelength resonator and coupling structure
978-1-4673-2185-3/12/$31.00 ©2012 IEEE

B. Filter parameter synthesize
The Chebyshev filter matrix synthesize method used in current design comes from reference [6] and [7]. The designed center frequency is 140GHz, bandwidth 13GHz, return loss lower than -20dB, 40dB rejection in 130GHz. 7-pole Chebyshev filter is selected. It is need to be noted that in millimeter and terahertz frequency waveguide which works in TE10 mode, transmission line dispersion will greatly influence the center frequency and the bandwidth. In this design, it uses method as follows to fix the primary design parameter, which could make filter parameter synthesized more accurately.
The guide wavelength of rectangular waveguide could be expressed as equation (1).
g 2 |
|
|
2 |
|
|
|
|
(1) |
||||||
|
|
|
|
|
|
|
|
|
|
|
|
|||
|
|
|
|
|
|
|
|
|
|
|
||||
|
|
|
|
2 |
m |
2 |
n 2 |
|||||||
|
|
|
|
|
|
|
|
|
|
|
|
|||
|
|
|
a |
b |
||||||||||
|
|
|
|
|
|
|
|
|
|
|
The fixed filter center frequency wavelength can be solved from equation (2), where λ0 is the center frequency, λ1 and λ2 is the up and down passband edge frequency wavelength.
1 sin( 0 ) g 2 sin( |
0 |
) 0 |
(2) |
|
|||
1 |
2 |
|
Then , the relative filter bandwidth can be got as equation (3), where f0,f1 and f2 is the center frequency, up and down passband edge frequency.
w |
2 1 |
|
f2 f1 |
(3) |
|
0 |
|
f0 |
|
The calculated center frequency wavelength under current parameter setting is λ0=2.8355mm. The calculated coupling coefficient K is: K01=K78=0.4975, K12=K67=0.2075, K23=K56=0.1496, K34=K45=0.1408, where m, n represent the serial number of the resonator. Every waveguide H ladder coupler can be represented as figure 3. The coupling coefficient and equivalent waveguide transmission line length can be calculated by mode matching method [8]. It is needed to note that, compared with thin coupler in lower frequency, the equivalent transmission length of coupler is significant, which should be added to the total length of resonator. The fillet, which is needed in mechanical fabrication, also will increase the electrical length of coupler.
|
|
|
|
|
|
|
|
|
|
|
|
|
|
|
|
|
|
|
|
|
|
|
|
|
|
|
|
|
|
|
Equivalent |
|
|
|
Equivalent |
|
|||||
Connected to |
transmission line |
Kmn |
|
transmission line |
Connected to |
||||||||
|
|
L0/2 |
|
L0/2 |
|||||||||
Resonator m |
|
|
|
Resonator n |
|||||||||
|
|
|
|
Coupling |
|||||||||
|
|
|
|
|
|
|
|
coefficient |
|
||||
|
|
|
|
|
|
|
|
|
|
|
|||
Figure 3. Equivalent circuit of H ladder coupler |
|
||||||||||||
|
|
|
|
|
TABLE I |
|
|||||||
|
|
|
SIMULATED COUPLER PARAMETER |
|
|||||||||
|
|
|
|
|
|
|
|
|
|
|
|
|
|
Serial number |
|
Coupling |
|
|
a1, mm |
|
|
L0, mm |
|||||
|
coefficient |
|
|
|
|||||||||
|
|
|
|
|
|
|
|
|
|
|
|
||
K01, K78 |
|
0.4975 |
|
|
|
1.107 |
|
|
|
0.7952 |
|||
K12, K67 |
|
0.2075 |
|
|
|
0.901 |
|
|
|
0.4733 |
|||
K23, K56 |
|
0.1496 |
|
|
|
0.843 |
|
|
|
0.3945 |
|||
K34, K45 |
|
0.1408 |
|
|
|
0.833 |
|
|
|
0.3819 |
|
3 |
|
|
Couple Coeff VS a1 |
|
|
|
|
||
Coeff(K) |
|
|
|
|
|
|
|
|
|
|
|
|
|
NO-Fillet |
|
|
|
|
|
|
|
|
|
|
|
|
|
|
|
|
|
|
Couple |
2 |
|
|
Fillet-0.150mm |
|
|
|
|
|
|
1 |
|
|
|
|
|
|
|
|
|
|
|
|
|
|
|
|
|
|
|
|
|
|
0 |
0.85 |
0.9 |
0.95 |
1 |
1.05 |
1.1 |
1.15 |
1.2 |
1.25 |
|
0.8 |
|||||||||
|
|
|
|
a1-Width of the Step WG(mm) |
|
|
|
|||
|
2 |
|
|
Equvilent Length VS a1 |
|
|
|
|||
Length(mm) |
|
|
|
|
|
|
|
|
|
|
1 |
|
|
|
|
|
|
|
|
|
|
Equvilent |
|
|
|
|
|
|
|
|
|
|
0 |
|
|
NO-Fillet |
|
|
|
|
|
|
|
|
|
|
|
|
|
|
|
|
||
|
|
|
|
Fillet-0.150mm |
|
|
|
|
|
|
|
-1 |
0.85 |
0.9 |
0.95 |
1 |
1.05 |
1.1 |
1.15 |
1.2 |
1.25 |
|
0.8 |
a1-Width of the Step WG(mm)
Figure 4. Simulated coupling coefficient and equivalent transmission length
The simulated coupling coefficient and equivalent transmission length is showed in table and figure 4. The difference with fillet and without fillet is also showed. The resonator consists of a certain length waveguide and two half coupler. The length of resonator waveguide can be get from equation (4), which is .
Lm |
|
0 |
|
L0m 1,m |
|
L0m,m 1 |
(4) |
|
|
|
|||||
|
4 |
2 |
2 |
|
The calculated data is : L1=L7=0.523mm, L2=L6=0.721mm, L3=L5=0.763mm, L4=0.768mm.
C. Total structure simulation
The resonators and couplers are cascade to be a total filter. It is simulated by mode matching method with mode number set to 30. Generally, mode matching method is more precision than finite element simulation tools like HFSS (high frequency structure simulator), which is restricted by the gridding density. Figure 5 showed the simulated filter S parameter, the center frequency is 140.0GHz, 3 dB bandwidth is 11.8GHz (134.0-145.8GHz), return loss is lower than - 18.1dB, -59.5dB rejection in 130GHz.
|
0 |
|
|
|
|
|
-10 |
|
|
|
|
(dB) |
-20 |
|
|
|
|
|
|
|
|
|
|
S parameter |
-30 |
|
|
|
|
-40 |
|
|
|
|
|
|
|
|
|
|
|
|
-50 |
|
|
|
S11 |
|
|
|
|
|
S21 |
|
-60 |
135 |
140 |
145 |
150 |
|
130 |
||||
|
|
|
Frequency (GHz) |
|
|
Figure 5. Simulated S parameter by mode matching method
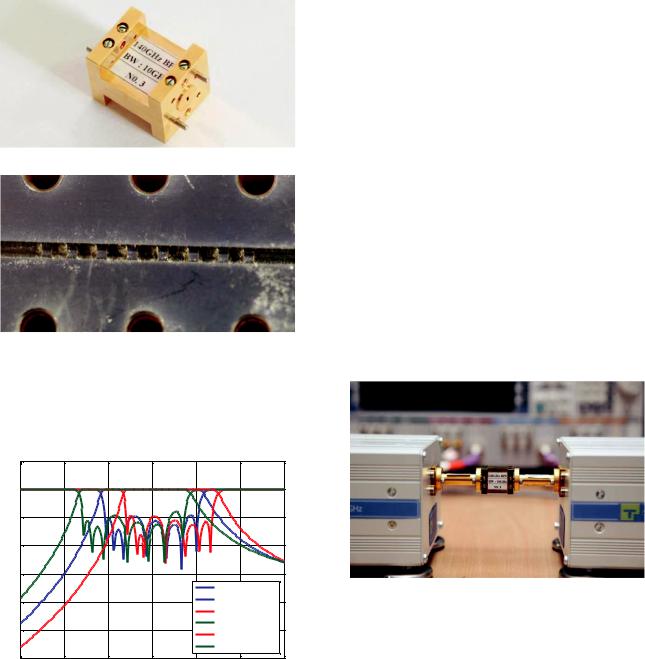
III. FABRICATION
The 140GHz bandpass filter is fabricated as split block by micrometer precision high speed CNC milling machines with tolerance +/-2.5um. Block material is copper. Simulation results indicate this tolerance will not affect filter performance significantly. The waveguide is split in E plane center, upper and lower block is connected by 8 screws to get perfect electrical contact. The block surface is plated with nickel-gold to avoid rusting. Figure 6 shows the picture of the final filter block. Figure 7 shows the waveguide structure of lower block.
Figure 6. Fabricated 140GHz bandpass filter
Figure 7. One side of filter waveguide split block
The milling precision could be well controlled by precision microscopy measurement and second compensating milling. Nickel-gold plating significantly affects the mechanical tolerance, which will thin the block when washing with acidic liquid and thicken the block when plating. The total waveguide mechanical variation will between -5 um to +15um.
|
20 |
|
|
|
|
|
|
|
0 |
|
|
|
|
|
|
(dB) |
-20 |
|
|
|
|
|
|
-40 |
|
|
|
|
|
|
|
S parameter |
|
|
|
|
|
|
|
-60 |
|
|
|
|
|
|
|
|
|
|
|
|
S11 T = 0um |
|
|
|
-80 |
|
|
|
|
S21 T = 0um |
|
|
|
|
|
|
|
S11 T = 10um |
|
|
-100 |
|
|
|
|
S11 T = -10um |
|
|
|
|
|
|
S21 T = 10um |
|
|
|
|
|
|
|
|
|
|
|
|
|
|
|
|
S21 T = -10um |
|
|
-120 |
130 |
135 |
140 |
145 |
150 |
155 |
|
125 |
||||||
|
|
|
|
Frequency (GHz) |
|
|
|
Figure 8. One side of filter waveguide split block
Nickel-gold plating will reduce the length of resonator and narrow the coupler equally. The resonance frequency and coupling coefficient also will alter synchronously. Simulation results indicate that the total performance of filter is more sensitive to the variation of resonator length than the variation of coupling aperture. So, it is possible to maintain certain passband performance as the filter center frequency changing when plating nickel-gold.
Figure 8 shows the simulated results of plating influence on filter. The block thickness is altering between -10 um to +10um (resonator length and coupling aperture altering between -20 um to +20um). Simulated data is listed in table
. The center frequency has been slightly changed 2GHz under 20um variation, about 1.4%. The relative 3dB bandwidth has been changed 9.6%.
TABLE II
SIMULATED PLATING INFLUENCE
Block |
Center |
3dB |
Maximum |
|
frequency, |
||||
thickness, um |
bandwidth, % |
return loss, dB |
||
GHz |
||||
|
|
|
||
+10 |
138.0 |
9.1 |
-15.8 |
|
0 |
140.0 |
8.3 |
-18.1 |
|
-10 |
142.1 |
7.5 |
-18.5 |
From the simulation and final measurement, it has been validated that the center frequency of 140GHz H ladder could be well controlled under carefully choosing plating technical parameters.
IV. MEASUREMENT
The filter S parameter has been measured from 110GHz to 170GHz by Farran FEV-06-TR-0003 D band vector network analyzer frequency extender. Power level is up to 0dBm. The dynamic range is about 60dB. The measurement environment picture is showed in figure 9.
Figure 9. Vector network analyzer S parameter measurement setup
The measured filter S parameter is showed in figure 10. The filter center frequency is 139.2GHz. The 3dB bandwidth is 11.6GHz (133.4GHz – 145.0GHz), 8.3% relative to center frequency. The minimum insertion loss is -1.0dB at 141.4GHz. Insertion loss increases to -2.5dB at 133.5GHz with return loss -21.9dB, increases to -1.5dB at 144.6GHz with return loss -

18.2dB. In theory, the minimum insertion loss frequency point is the filter resonating frequency, insertion loss will increase when leaving from the resonating frequency. In lower frequency, it is not very significant. But in millimeter and terahertz band, it can easily be observed. The entire passband return loss is lower than -16.4dB. The stopband rejection is - 48.1dB at 130GHz, 6.6% away from the center frequency.
Figure 10. Measured filter S parameter
The difference between measured and simulated data is showed in figure 11. 7 return loss resonating point could be observed clearly. The center frequency is 0.8GHz lower than simulated data, about 0.6% leaving from 140GHz. Bandwidth is 0.2GHz lesser, about 98.9% of design target. Return loss is 1.7dB higher than simulation. The measured data coincide well with simulation results.
|
0 |
|
|
|
|
|
-10 |
|
|
|
|
(dB) |
-20 |
|
|
|
|
|
|
|
|
|
|
S parameter |
-30 |
|
|
|
|
-40 |
|
|
|
|
|
|
|
|
|
|
|
|
-50 |
|
|
Measured S11 |
|
|
|
|
Measured S21 |
||
|
|
|
|
Simulated S11 |
|
|
-60 |
|
|
Simulated S21 |
|
|
135 |
140 |
145 |
150 |
|
|
130 |
||||
|
|
|
Frequency (GHz) |
|
|
In this paper, the research of 140GHz bandpass filter are presented. The filter is based on 7-pole waveguide H ladder structure, simulated by mode matching method, fabricated based on micrometer precision high speed CNC milling machines. Measured data indicates the filter center frequency is 139.2GHz. The 3dB bandwidth is 11.6GHz, 8.3%. The minimum insertion loss is -1.0dB. The passband return loss is lower than -16.4dB. The stopband rejection is -48.1dB at 130GHz. It has been successfully used in 140GHz transmitter and receiver of THz communication system of CAEP.
ACKNOWLEDGMENT
The works reported in this paper were supported by Terahertz Research Center, Institute of Electronic Engineering, China Academy of Engineering Physics. The authors would like to thank Prof. Zhang Jian and Prof. Xiao Shiwei of Institute of Electronic Engineering, China Academy of Engineering Physics, for their direction in circuit design and fabrication.
REFERENCES
[1]Wang Cheng, Lin Changxing, Deng Xianjin and Xiao Shiwei, “140GHz High Data Rate Wireless Communication Technology Research”, Journal of Electronics & Information Technology, vol. 33, no. 9, pp 2263-2267, Sept. 2011.
[2]R. Dickie, R. Cahill, V. Fusco, H. S. Gamble and N. Mitchell, “THz Frequency Selective Surface Filters for Earth Observation Remote Sensing Instruments”, IEEE Trans. Terahertz Science and Tech., vol. 1, no. 2, pp 450-461, Nov. 2011.
[3]M. Euler, V. F. Fusco, R. Cahill, and R. Dickie, “325 GHz single layer sub-millimeter wave FSS based split slot ring linear to circular polarization convertor,” IEEE Trans. Antennas Propag., vol. 58, no. 7, pp. 2457–2459, Jul. 2010.
[4]Yuan Li, P. L. Kirby and J. Papapolymerou, “Silicon Micromachined W-Band Bandpass Filter Using DRIE Technique”, 2006 European Microwave Conference, Manchester UK, Sep. 2006, pp 1271-1273.
[5]S. V. Robertson, L. P. B. Katehi and G. M. Rebeiz, “Micromachined W- Band Fliters”, IEEE Trans. Microwave Theory Tech., vol. 44, no. 4, pp 598-606, April. 1996.
[6]R. J. Cameron, “Advanced Coupling Matrix Synthesis Techniques for Microwave Filters”, IEEE Trans. Microwave Theory Tech., vol. 51, no. 1, pp 1-10, Jan. 2003.
[7]R. J. Cameron, “General Coupling Matrx Synthesis Methods for Chebyshev Filtering Functions”, IEEE Trans. Microwave Theory Tech., vol. 47, no. 4, pp 433-442, April 1999.
[8]Zhai Yang Wen, Shi Xiao Wei and Zhao Yong Jiu, “Full-wave analysis and rigorous design of the waveguide transverse iris band-pass filter”, Journal of Xidian University, vol. 34, no. 4, pp 521-525, Aug. 2007.
V. CONCLUSION
Figure 11. Comparison between measured and simulated data
The difference between measured and simulated data is caused by many reasons. First, the metal loss and surface roughness affect the transmission loss. Second, the fabrication (milling and plating) error is added to primary design. Third,
the simulation error cannot be ignored. Finally, the measurement system has error about +/-0.5dB.