
диафрагмированные волноводные фильтры / cf0541c4-5956-4bdb-8f5b-5640ba5e8aa4
.pdf
AHigh Stop-band Suppression W-band Waveguide E-plane Filter for millimeter-wave applications
W. J. Wu, R. Zhang, X. X. Fan
School of physics and electronics, University of Electronic Science and Technology of China Chengdu, 610054, P. R. China
wenxiangtaoli@163.com
Abstract-E-plane filter have been widely used in microwave and millimeter-wave circuits for its high Q value, low loss and easy to realized. In this paper, a low-order W-band Wave guide E-plane fin-line band pass filter are used to provide high harmonics suppression for millimeter-wave devices such as six frequency multiplier. The equivalent circuits of the filter are analyzed and a simple CAD method is introduced for E-plane band pass filter synthesis. The experimental result show that the insertion loss is less than 3 dB, and the center frequency is 93 GHz with a bandwidth of 2 GHz and the suppression is greater than 42dB near 88 GHz, which means the fifth harmonic (near 78 GHz) of the six frequency multiplier (operating at 94 GHz) are suppressed effectively.
I.INTRODUCTION
High selectivity low-cost and low-loss W-band filters become increasingly critical in the 3-mm region of millimeter wave systems. E-plane filter have been widely used in microwave and millimeter-wave circuits because they have many favorable properties such as simple structure, low pass band insertion loss, easy to mass production. Thereafter, except for all-metal inserts [1]-[4], it expanded to single-ridge finline [5], double-ridge finline [5], large gap finline insert structures [6].
Although E-plane fin-line filters have higher machining precision than the metal-insert type, they are still difficult to suppress the frequency points which are close to the passband [7]. Pure metal inserts placed in the E-plane of rectangular waveguides without any substrates achieve low-loss designs. In this paper, we present a low-order E-plane metal sheet inserted filter with high-rejection and low-loss characteristics. A simple CAD method is introduced for E-plane bandpass filter synthesis based on the analysis of the equivalent circuits of the E-plane metal sheet. By properly choosing the dimensions of the metal sheet and optimizing the cavities length between the metal sheet, the suppression level at the unwanted frequency points near the passband can be achieved without disturbing the passband performance.The measured results present highrejection characteristics while maintaining low cost and simple
structure
II.E-PLANE METAL SHEET FILTER DESIGN
A. Equivalent circuit analysis for E-plane metal sheet
The structure of E-plane filter is shown in Fig. 1, It is comprised of a rectangular wave guide and all-metal inserts placed in the E-plane of a rectangular waveguide along the waveguide axis. A single metal sheet is shown in Fig. 2a, And
Figure 1. The structure of Wave guide and layout of the proposed filter
Figure 2. Equivalent circuit of single E-plane metal sheet
it is equal to a T-type equivalent inductor network in Fig. 2b, Assuming a TE10-type incident wave, the exited fields are composed of the TEn0-type waves. All of higher modes are localized around the E-plane sheets. They are evanescent modes so only dominant mode are taken into consideration. By using the method mentioned in [5], in the case of short circuit and open circuit at the central plane T0 of the metal sheet, we can find the relationship between the normalized input
admittance Y(Yshort, Yopen) and the impedance X(Xs, Xp) as follow
Y |
short |
= − |
1 |
(1) |
|
jX s |
|||||
|
|
|
|||
|
|
|
|
Yopen = |
1 |
|
(2) |
|
j(2X p |
+ X s ) |
|||
|
|
Let the scattering matrix elements of the fundamental mode be named S11, S12, S21, S22. According to two-port network theory, we can get
978-1-4673-8983-9/16/$31.00 ©2016 IEEE
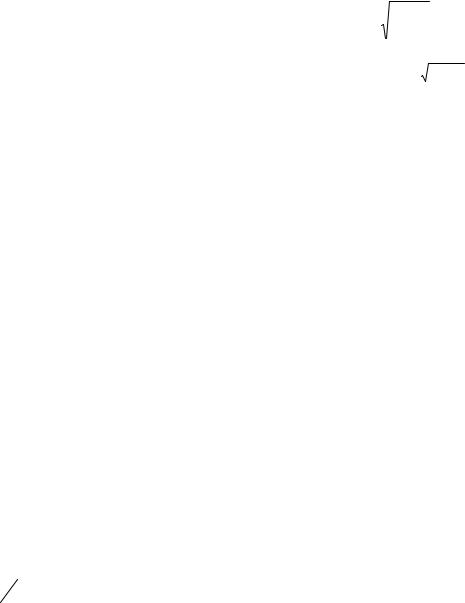
jX s = |
1− S12 |
+ S11 |
(3) |
|||
1− S11 |
+ S12 |
|||||
|
|
|||||
jX p = |
2S12 |
|
(4) |
|||
(1− S |
|
)2 |
− S 2 |
|||
|
11 |
|
12 |
|
Where Xs, Xp are both normalized by waveguide characteristic impedance. Since the structure can be simulated easily by commercial 3D EM simulation software, such as HFSS. Then the S-parameters of the E-plane sheet could be obtained easily and then we get series impedance Xs, and parallel impedance Xp with (3) to (4).
A shunt inductor with inductance far less than the transmission line characteristic impedance could be characterized by the equivalent impedance inverter with a pair of negative electrical length transmission line [5]. In fact, the length of air cavity Li is about half-wavelength which act as a resonator while the metal sheet insert to realize a K inverter. So the E-plane sheet is transformed into the equivalent circuit in Fig. 2c, In the transformation from T-type network to K inverter, the parameters have relationships as follow:
Ki−1,i |
|
1 |
φi + arctan X si ) |
|
||||||||
= |
tan( |
(5) |
||||||||||
|
|
|
|
|
|
2 |
|
|
|
|
|
|
li = |
|
|
|
1 |
|
|
|
|
λg |
|
||
π + |
|
(φi + φi+1) |
× |
|
|
(6) |
||||||
2 |
2π |
|||||||||||
|
|
|
|
|
|
|
|
|
φi |
= − arctan(X pi |
|
+ X si ) − arctan(X si ) |
(7) |
||||||||
θ |
|
= |
|
2π |
L − |
1 |
(φ |
|
+ φ |
|
) |
(8) |
|
|
λg |
2 |
|
|
|||||||
|
i |
|
|
i |
|
i |
|
i+1 |
|
|
||
λg |
|
= |
|
|
λ0 |
|
|
|
|
|
(9) |
|
|
1− λ0 |
|
2 |
0.5 |
|
|
||||||
|
|
|
|
|
|
|
||||||
|
|
|
|
|
|
|
|
|
|
|
|
|
|
|
|
|
|
2a |
|
|
|
|
|
||
|
|
|
|
|
|
|
|
|
|
|
|
where Ki-1,i, Xsi, Xpi are both normalized by wave guide characteristic impedance.
B. A simple CAD method for filter synthesis
Since K and θ are determined by operating frequency and the width of metal sheets. Once we know the series impedance Xs and parallel impedance Xp correspond different width of metal sheet, we can get the parameters K and θ easily by (5) to
(9) and then we can construct a function mapping as follow:
g(width, fre) → h(K,θ ) |
(10) |
According to the design target of filter ,we can calculate K value as follow:
K0,1 = Kn,n+1 |
= |
πFBW |
|
(11) |
||||
2g0 g1 |
|
|||||||
|
|
|
|
|
|
|||
Ki,i+1 |
|
i=1...n−1 |
= |
πFBW |
1 |
(12) |
||
|
||||||||
|
2 |
|
|
gi gi+1 |
||||
|
|
|
|
|
|
|
||
FBW = ω 2 − ω1 |
|
(13) |
||||||
|
|
ω 0 |
|
|
|
|
|
Then we can construct a new function mapping as follow:
h(K,θ ) → g(width, fre) |
(14) |
The commercial EM software HFSS was used for EM simulation to get the S-parameters of a certain width sheet. And then we can calculate its K value use (3) to (13). We can use HFSS to scan for a variety of widths of sheet, compare the calculated K value with ideal K value which is given by filter prototype and find the optimum width value eventually. So the dimension parameters of the filter structure can be obtained using (14) with HFSS parameters sweep rather than solving complicated equation.
C. Initial dimension parameters design
In our design, the rectangular wave guide for the E-plane filter working in W-band is WR10 having width a = 2.54 mm, height b = 1.27 mm and the thickness of inserted metal sheet is 0.1 mm. A single E-plane inserted metal sheet model is established in HFSS are shown in Fig. 3, and the simulation frequency range from 85 to 105 GHz. The width of the E-plane sheet adopts 40 points in equal intervals from 0.05 mm to 2.0 mm. The single E-plane inserted metal sheet simulation results (simulated at 94 GHz) are also shown in Fig. 3, Which demonstrate the function relationship between sheet width and impedance invert K, we could find the the optimum width to match the required K value. Then we could get the value of θ as it only relative to K and frequency. The value of θ is to determine the length of wave guide between two adjacent E- plane sheets.
According to the above method we designed a three-order W-band E-plane sheet inserted filter, operating at center frequency 94 GHz with 2 GHz bandwidth are specified for the filter design. All the dimension parameters are summarized in Tab. 1.
TABLE I The Design Parameters of Inserted Metal Sheet
BW |
w1=w2 |
w3=w4 |
L1=L3 |
L2 |
2 GHz |
0.35 mm |
1.35 mm |
1.50 mm |
1.49 mm |
|
|
|
|
|
III. SIMULATED AND MEASURED RESULTS
With accuracy optimization of electric magnetic simulation software HFSS, the final simulated results are shown in Fig. 4, in which a sharp rejection on the lower side can be clearly observed. The suppression is about 52 dB at 88 GHz. The proposed filter (three-order) has been fabricated and measured.The actual picture of the filter is shown in Fig. 5, and

the measured results are also depicted in Fig. 5. The minimum measured insertion loss is 2.0 dB in the pass band from 92 to 94.5 GHz. The return loss is greater than 10 dB from 92 to 94.5 GHz and the return loss is greater than 21 dB at 94 GHz. The suppression is more than 42 dB below 88 GHz. In addition, it could also provide over 80 dB suppression at the fifth harmonic (near 78 GHz) of the six frequency multiplier which operating at 94 GHz.
It can be found that there is some difference between the measured and the simulated about the mid-band frequency. This is mainly introduced by the limited dynamic range of the VNA operating in this frequency band and the manufacturing error about the width and thickness of inserted metal sheet. The finite sheet thickness considerably influencing passband ripple behavior and mid-band frequency, as has already been demonstrated in [8].We can obtain better experimental results by improving the manufacturing precision.
presented in this paper. Experiment results agree well with the analysis and simulation results. Measured results show that the insertion loss is about 2dB with about a 2.1% fractional bandwidth at 93 GHz, and the suppression achieves over 42 dB at 88 GHz which is only 5 GHz lower than the center frequency. So the filter could be used to provide high suppression for millimeter-wave devices such as six frequency multiplier.
Figure 3. Plot of normalized impedance inverter K versus septum width with frequency at 94 GHz
Figure 4. Simulated results of the W-band E-plane all-metal inserts filter.
IV. CONCLUSION
A W-band E-plane wave guide filter employing a conventional metal inserted structure is proposed. Base on the analysis of the equivalent network and its transformation, a simple CAD method to acquire the dimension of the filter is
Figure 5. Measured results of the W-band E-plane all-metal inserts filter.
ACKNOWLEDGMENT
The authors wish to thank J. Xu, X. C. Zhang and G. P. Li of for useful academic advising and provide the VNA for the measurement of filter.
REFERENCES
[1]Y. Konishi, K. Uenakada, “The design of a bandpass filter with inductive sheet-planar circuit mounted in waveguide,” IEEE Trans. Microwave
Theory Tech., vol. 22, pp. 869–873, October 1974.
[2]R. Vahldieck, J. Bomemann, F. Amdt, D. GrauerhoJz, “Optimized waveguide E-plane metal insert filters for millimeter-wave applications,”IEEE Trans. Microwave Theory Tech., vol. 31, pp. 65-69, January 1983.
[3]J. S. Hong, “Design of E-plane filters made easy ” , IEEE Proceedings Microwaves, Antennas and Propagation , vol. 136, Issue 3, pp. 215-218, June 1989.
[4]V. Postoyalko, D. S. Budimir, "Design of waveguide E-plane filters with all-metal inserts by equal ripple optimization", IEEE Trans. Microwave Theory Tech., vol. 42, no. 2, pp. 217-222, February 1994.
[5]Y. C. Shib, T. Itoh, I. Q. Bui, “Computer-aided design of millimeter-wave
E-plane filters,”IEEE Trans. Microwave Theory Tech., vol. 31, no. 2,
pp. 135-141, February 1983.
[6]J. Uher, J. Bornemann, U. Rosenberg, Waveguide Components for Antenna Feed Systems: Theory and CAD, Artech House, Boston, 1993.
[7]Z. B. Xu, J. Guo, C. Qian, W. B. Dou, “Broad-band E-plane filters with improved stop-band performance,” IEEE Microwave and Wireless
Components Letters, vol. 21, no. 7, pp. 350-352, July 2011
[8]R. Vahldieck, J. Bomemann, F. Amdt, and D. GrauerhoJz, “Optimized waveguide E-plane metal insert falters for millimeter-wave
applications,”IEEE Trans. Microwave Theory Tech., vol. 31, no. 1, pp. 65-69, January 1983.