
книги / FISMA and the risk management framework the new practice of federal cyber security
..pdf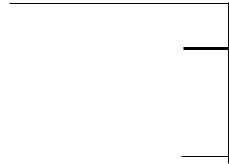
Mechanical analysis of the scratching of metals and polymers at moderate and large strains |
47 |
Scratching ofPolycarbonate
It appears clearly on the side view of the scratch (Fig. 21) that no such transition occurs in polycarbonate even for the lowest cone angle 20 deg; the flow pattern is always of ploughing type with a frontal edge whose height does not vary significantly with 8. The origin of this difference with steel however requires some discussion because the simulations related to polycarbonate have been performed with a friction coefficient Ji=0.3 whereas the ones related to steel with zero friction. In addition the rheology of the two materials presents very great differences (Table 2).
+We have already briefly discussed the influence of the strain rate sensitivity which does not seem very important(§ 4) because the strain rate exponent m is rather low (0.052).
+For 8=70.3 deg the apparent index of indentation of polycarbonate is rather low:
X=(E/cr1)cot8-8.4; examination of the contact surface demonstrates effectively that there exists a rear contact surface and so the apparent friction coefficient is J!o-0.527 a value little lower than the value provided by the relation (21) : 0.546; so these results are not very different from the results related to the elastic perfectly plastic solids studied in § 5 for the same value of the indentation index. However we observe in Fig. 21 the formation of a frontal edge which is not in agreement with the results of Fig. 9; the real scratch hardness is effectively lower. The friction level explains these facts: we have observed that for polycarbonate scratched by a conical indenter with a semiapical angle 30 deg and having a spherical tip ("rounded indenter A") the frontal edge height increase with friction as for the workhardened steel (Fig. 14) [29].
+Finally, we have very clearly demonstrated that the very great stability ofthe ploughing flow for acute cones is due to the high workhardenability of the polycarbonate: if we limit the increases in flow stress at a plastic strain level 1.5, which corresponds to 3 times increase of the strength according to the relation ( 16) and the values of table 2, we observe the formation of a chip during scratching by the "rounded indenter A" under the same friction coefficient !!=0.3, despite the fact that this kind of indenter is less acute than the perfect cone with the same semiapical angle [37].
Fig. 21. Influence of the semiapical angle 8 of a conical indenter on the scratch geometry (side view; plane x=O) ofpolycarbonate (Ee-4.25 %; hg=0.5) (!!=0.3)
At this time, it is very instructive to observe the groove profile under load (plane y=O) and its plastic strain map (Fig. 22) for the four cone angles. They are very significantly different from those related to steel (Fig. 20). First a residual groove exists in all cases, but it is very much
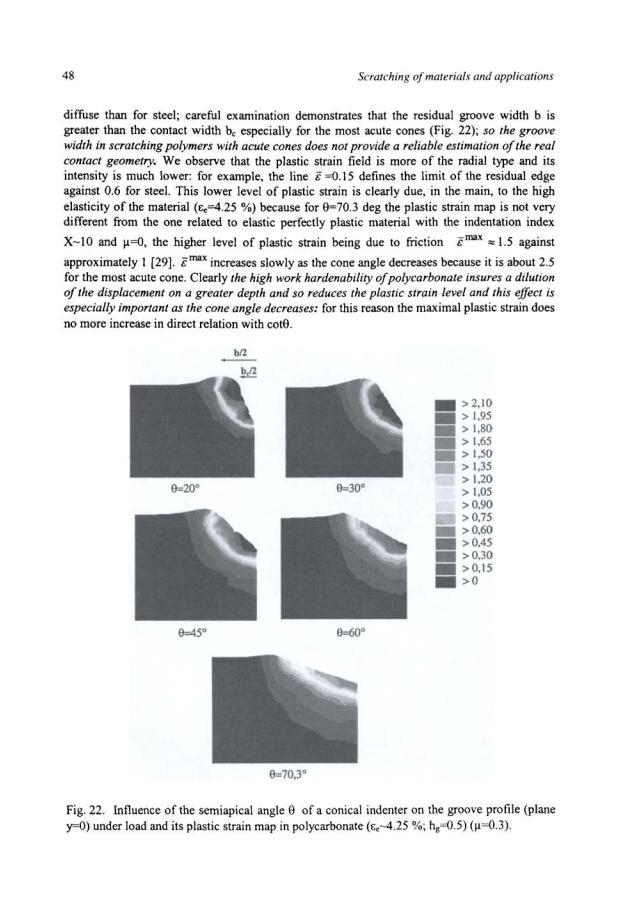
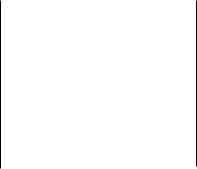
Mechanical analysis of the scratching of metals and polymers at moderate and large strains |
49 |
For 9=70.3 deg the apparent fiiction coefficient J.lo is well predicted by the relation (21) as we have previously noticed, but due to the complex evolution with the cone angle of the flow and the contact geometry this relation predicts less and less well J.lo as the cone angle decreases; the discrepancy being maximal for the most acute cone 9= 20 deg. In this case, the numerical simulation provides the values llo=l ()l=O) and 1.26 ()1=0.3) against J.lo=l.74 (J.l=O) and 2.04 (J.1=0.3) for the relation (21). So the contribution of the adhesion term remains correct, the discrepancy being due to the ploughing term which is more and more overestimated as 9 decreases because the rear contact surface increases as the cone angle decreases: the elastic strain increases due to the increase in the contact pressure as we see it below. The fact that the flow lines remain in planes parallel to the plane x=O in first approximation as suggested by the adhesion term of the apparent fiiction coefficient is another confirmation of the increasing influence of elasticity which restricts the deviation of the flow lines from the scratch speed.
10 |
|
|
|
H /a |
|||
|
|||
|
v· |
1 |
|
8 |
\ |
|
|
|
|
6 |
'\ |
|
|
|
|
|
|
|
|
|
|
|
|
|
|
|
|
|
|
|
\ |
|
|
|
|
|
4 |
|
|
|
|
|
H |
/a |
|
|
|
|
|
|
|
1 |
||
|
|
|
|
|
|
|
1/2 |
|
2 |
|
|
|
|
|
|
|
|
|
|
|
|
|
|
e (deg) |
||
0' ----- ' ---- ' ---- '' ----- ' ---- ' ------- ' |
||||||||
20 |
30 |
40 |
50 |
60 |
70 |
|
80 |
Fig. 23. Influence of the semiapical angle 9 of a conical indenter on the scratch hardness of polycarbonate (~:.-4.25%; hg=O.S) (J.1=0.3).
Figure 22 provides the evolution of the scratch hardness with the cone angle. Due to the high hardenability of polycarbonate and despite the decrease in contact pressure observed for perfectly plastic steel as 9 decreases (Fig. 19), Hs increases steadily and attains a very high value -10 cr1 for 9=20 deg. On the contrary the apparent scratch hardness Hsi/2 which is higher than Hs for the blunter cones increases initially as e decreases, is maximal -3.5 <Jj for 9=45 and 30 deg and then decreases a little as observed in experiments [8]. This difference in evolution of the two scratch hardness values is due to the difference between the residual groove width and the effective contact width (Fig. 22). These results explain the high scratch resistance of polymers which can be improved by changing their structure and so increasing their strain hardening coefficient hg [29, 37].
Discussion ofthe Results and Nature ofFurther work
It is now time to perform an estimation of the limits of such a work on scratching of metals and polymers.
First, we shall recognise again that because metals have low elasticity, they undergo high plastic strain in scratching, and so the level of numerical error is certainly higher than for high elasticity materials such as polymers. In addition this numerical error increases certainly as the cone angle
50 |
Scratching of materials and applications |
decreases or the friction increases. Nevertheless the comparison with available experimental results demonstrates clearly that the main conclusions are still valid. It remains to perform more accurate comparisons by taking into account more detailed rheological laws. For metals this task seems relatively easy because their elastic strains remain in all cases very low and well described by the classical theory of elasticity. Their flow stress at constant temperature can be described in a large range of strain and strain rate by power laws:
(22)
Notice that such a rheological behaviour induces some reduction in the plastic strain level with respect to perfectly plastic solids and so reduces the numerical error level. Nevertheless some difficulties remain and require some consideration. The increase in temperature T could not be negligible and in this case the influence ofT on the parameters of (22) must be included, the energy equation must be integrated simultaneously with the appropriate boundary conditions.
So the problem becomes rather complex. But it is interesting to remark that the intensity of the thermal effect can be characterised by the Peclet's number Pe:
Vb |
2 i |
strain rate &.=-v |
(23) |
Pe=- =b |
- with |
b |
|
K |
(K ) |
lthermal diffusivity K |
|
The loss of the mechanical energy involved in the plastic strain of the material by heat conduction restricts more and more the increase in temperature as Pe decreases. So reducing in proportion the penetration depth h (and so the groove width b) and the scratch velocity V in order to maintain the order of magnitude of the strain rate of the material permits to reduce the thermal effects without changing the level of strain and strain rate: we thus observe all the interest of the nanoscratch testers which can impose very low penetration depths. For example for a steel (K- 10 mm2.s-1) with h=l )liD and V = 1 )lm.s·1, i"' 1 s·1 and Pe- 10-7 ! For this very low value of Pe the scratching can be considered as isothermal with a very good approximation. Despite their very much lower thermal diffusivity (K- 0.1 mm2.s-1) this approximation remains valid at this scale of testing for polymer. So the nanoscratch test appears as a mean to study large strains of metals and polymers at high strain rates under quasi-isothermal conditions.
However due to the inhomogeneous state of strain and strain rate interpretation of the test requires at present time numerical simulations.
We must notice however that at these small penetration depths rheological description of even metals is not so easy as flow stress is affected by the size effects due to the discontinuous character of the plastic deformation which involves the movement of dislocations [38]. For polymers the problem appears as still more complex because their rheological behaviour even at macroscopic scale is very complex [31, 39-41].
One question emerges: Is the effect of the plastic strain on polymers well described by relation (16) ? At present time, the answer is "yes". We have demonstrated that saturation in the hardening produces for acute cones the formation of a chip which is not observed experimentally. Another interesting validation is provided by the experimental results obtained by scratching another thermoplastic polymer, the poly(methylmethacrylate) (PMMA), by the

Mechanical analysis of the scratching of metals and polymers at moderate and large strains |
51 |
Berkovich pyramid [42]. The scratchings are performed on a nanoindentation device on which it is possible to scratch the material with a rather high normal force (which corresponds to a penetration depth h-1.4 Jlm, the scratching velocity being V-10 Jlm.s·') and after to measure the residual groove profile and the local nanohardness of the material with a much lower normal force and penetration depth (for a penetration depth h-30 nm). So it appears that the residual groove depth hr (Fig. I) is very low: 0,1 h and 0,23 h for respectively edge and face scratching; in parallel the residual groove height hb increases. The nanohardness before scratching is about Ho-0.3 GPa; the nanohardness of the material of the tops of the residual groove is equal to Hres-0.5 GPa and 0.7 GPa for edge and face scratching respectively. It is possible to deduce from such values an estimation of the equivalent residual plastic strain of the material with the following relation suggested by the rheological equation (16):
- |
|
1 |
l (Hres) |
(24) |
&res- |
|
hg |
n |
|
|
|
Ho |
|
The rheological law ofPMMA is very similar to the one ofpolycarbonate (it has an equal value for the workhardening coefficient h8 [29]). Application of relation (24) suggests that the residual plastic strain attains respectively 1 and 1.3, values in agreement with the results of the numerical simulation of edge and face scratching with Berkovich pyramids [29]. In addition, the numerical simulation demonstrates that the plastic strain in the groove centre for face scratching is still higher about 1.8. So although the residual penetration depth is very low the residual mechanical properties of the polymer are well affected as described by the relation (16).
Finally it is instructive to try to evaluate the elastic strain component, a representative plastic strain and a total scratch strain starting from the numerical values of the scratch hardness of polycarbonate. For this purpose we propose the following relations:
el Hs
&ap=E
(25)
We choose a constrain factor C=l.5, independent on the cone angle, a debatable assumption, but in fact the conclusions that we can draw from the numerical application of these relations (Table 3) remain valid if we change this value. We add the evaluation &ap =1.5 cote for two reasons:
Firstly this expression permits to understand the evolution of the apparent scratch hardness of metals with the cone angle (power hardening law (23) with m='O), secondly the relation (25) does not provide a reliable evaluation of the apparent plastic strain for the bluntest cone where the strain hardening has no significant influence on the scratch hardness according to (16) as we can easily verifY it.
52 |
Scratching of materials and applications |
From the results obtained with these formulas (Table 3) we can draw the following conclusions:
• First the elastic component of deformation increases greatly as 9 decreases and attains 41 % for 9=20 deg; clearly the description of such a (visco)elastic deformation would require more elaborate models than the classical theory of(small) elastic strain.
•We see in addition that for 9=60 deg, both the evaluations of the apparent plastic strain Cap are in rather good agreement and so the representative plastic strain proposed for
metals whose hardenability is very much lower seems reliable for 9=70.3 and 60 deg.
•But for the most acute cones 9=45-30-20 deg which tend to induce higher plastic strains it is the relation (25) which provides the best estimation because the high hardenability
of polymers restrains the level of plastic strain as previously seen (see Fig. 22).
•For the three bluntest cones 9=70.3-60-45 deg the part of the elastic component is nearly constant -10 % whereas it increases dramatically for the most acute cones 9=30-20 deg. So at present time it is rather difficult to propose a simple model of scratching of polymers.
Table 3. Evaluation of the elastic and plastic component (the most reliable estimation is in bold character) of deformation deduced from the results of the numerical simulation of scratching of polycarbonate (C=l.5- tap =1 s·').
e(deg) |
70.3 |
60 |
45 |
30 |
20 |
el |
0.064 |
0.089 |
0.138 |
0.29 |
0.41 |
Eiap |
|
|
|
|
|
E:ap(25) |
0 |
0.82 |
1.25 |
1.74 |
1.93 |
|
|
|
|
|
|
1.5cote |
0.53 |
0.86 |
1.5 |
2.6 |
4.12 |
&sap- |
0.6 |
0.9 |
1.4 |
2 |
2.3 |
|
|
|
|
|
|
Ei~~ I &sap (%) |
10,7 |
9.7 |
10 |
14.3 |
17.5 |
|
|
|
|
|
|
|
|
|
|
|
|
Another limitation of our rheological description of polymers is the assumption of isotropic hardening. After reversing the stress for example in shear, yield occurs in fact for lower stress [31]. Such a phenomenon certainly plays a role during the recovery at the rear part of the contact: our calculations overerestimate greatly the residual penetration depth hr (Fig. I). Recently Kermouche et. al. [43] have demonstrated by numerical simulation of the scratching with a sphere at low penetration depth that the recovery increases greatly for a material having kinematic hardening.
Finally, the real conical indenters have always a tip defect which is very commonly assumed spherical. The study of the influence of this indenter tip radius R which can be significant in nanoscale testing is to our knowledge well developed in indentation but not in scratching. It is easy to apply the current way usually used in analysis of indentation in order to define some equivalence between conical and spherical indenters [19]. To a first approximation, the spherical tip reduces the effective attack angle 13 of the indenter for a penetration depth h lower than the spherical cup height h*, so we have:
Mechanical analysis of the scratching of metals and polnners at moderate and large strains |
53 |
||
tan fJ - -a - H- ~ X - |
- E~- h < h* =R(l- sm. B) |
(26) |
|
R |
R |
ay R |
|
So the increase in penetration depth is equivalent to an increase in the indentation index. This fonnulation provides a simple way for applying the present results to real indenters. Evidently, further work is required in order to evaluate the reliability of this equivalence law and refine it.
CONCLUSIONS
Despite some limitations in the comparisons with the experimental work, the present work demonstrates the power of the numerical simulation in order to develop the mechanics of the scratch test of materials.
+First we propose a very simplified 3D cone scratching model based on an analogy with 2D wedge scratching which provides some very simple explanations of the results of the numerical simulation in the case of low elasticity material (metals): level of volume average plastic strain, influence of friction on contact pressure and influence of the cone angle on the flow pattern and the scratch hardness.
+We state precisely the similarities on the influence of elasticity of materials in indentation and scratching: the real contact pressures are nearly equal in indentation and scratching of elastic-perfectly plastic material under zero friction for the axisymmetrical cone equivalent to the Vickers pyramid.
+We also elucidate the influence of the indentation index on the rear contact surface, a problem specific to scratching: As a first approximation, the contact surface is limited partly by a circle; as the indentation index X increases, i. e. for less and less elastic materials, the contact radius increases for a given penetration depth whereas the rear contact angle decreases and this decrease induces an increase in the ploughing friction.
+Numerical simulations demonstrate that scratching induces higher plastic strain than indentation. They validate many assumptions commonly adopted in the analysis of scratching of metals: contact geometry (half of a circular disk), relation between cone angle, friction and apparent friction coefficient.
+We confinn also some other common assumptions about the main differences between indentation and scratching especially on the influence of friction: For low elasticity materials and blunt indenter the increase in friction produces a reduction in contact radius and an increase in contact pressure in indentation whereas the inverse occurs in scratching. The increase in friction produces mainly an increase in the frontal edge; so the frontal part of the contact is limited by half of an ellipse whose minor axis length is nearly constant and the apparent scratch hardness does not vary.
+Finally, we demonstrate that low cone angles induce a composite flow cutting-ploughing in scratching elastic perfectly plastic materials as workhardened metals whereas the high hardenability of polymers stabilises the ploughing flow till the lowest cone angle investigated (20 deg), restrains the plastic strain and increases greatly the contact pressure. These results explain the high scratch resistance of polymers which can be improved by changing their structure and so increasing their strain hardening coefficient hg.
54 |
Scratching of materials and applications |
ACKNOWLEDGEMENTS
Essilor INTL Coatings Research and Development is acknowledged for its interest for the results and its financial support for part of this work. We wish especially to express our thanks to Dr. G. Hochstetler and M.A. Jimenez for hepful discussion during the course of this work.
REFERENCES
1.de Reaumur, R. A. F. L' Art de Convertier le Fer Forge en Acier, Paris 1722, as referred in Petty E. R. (1971). In Measurements of mechanical properties, Techniques of metal research, Vol V Part 2, pp.158-220, Bunshan R. F. (Ed), J. Wiley & Sons, New York.
2.Tabor D. (1954) Proc. Phys. Soc. B67, 249.
3.Bowden P. and Tabor D., ( 1950-1964). The friction and lubrication ofsolids, Clarendon Press, Oxford.
4.Brookes C. A., Green P., Harrison P. H. and Moxley H. B. (1972). J. Phys. D: Appl. Phys. 5, 1284.
5.Williams J. A. (1996). Trib. Int. 29, W8, 675.
6.Mulhearn T. 0. and Samuels L. E. (1962). Wear 5, 478.
7.Buttery 0. and Archard J. F. (1970-71). Proc. Instn Mech. Engrs 165 (43/71) 537.
8.Briscoe J., Pelillo E. and Sinha S. K. (1996). Polym. Eng. Sci. 36, 2996.
9.NiB. Y. and Le Faou A. (1996). J. Mater. Sci. 31,3955.
10.Jardret V., Zahouani H., LoubetJ. L. and Mathia T. G. (1998). Wear 218, 8.
11.Gauthier C. and Schirrer R. (2000). J. Mater. Sci. 35, 2121.
12.Bertrand Lambotte P. (2001). Sur les mecanismes de rayure des vernis definition automobile, These de Doctorat en Mecanique, Ecole Centrale de Lyon.
13.Gauthier C., Lafaye F. and Schirrer R. (2001 ). Trib. Int. 34, 469.
14.Childs T. H. C. (1970). Int. J. Mech. Sci. 12, 393.
15.De Vathaire M., Delamare F. and Felder E. (1981). Wear 66, 55.
16.Gilormini P. and Felder E. (1983). Wear 88, 195.
17.Torrance M.A. (1988). Wear 123, 87.
18.Tabor D., (1970). Rev. Phys. Techno/., 145.
19.JohnsonK. L. (1970).J. Mech. Phys. Solids 18,115.
20.Hill R., Storakers B. and Zdunek A. B. (1989). Proc. R. Soc. Land. A 423,301.
21.Challen J. M. and Oxley P. L. B. (1979). Wear 53, 229.
22.Lee E.H. and Shaffer B.W~(1951) J. Appl. Mech. 18, 405.
23.Lebouvier D., Gilormini P. and Felder E. (1985) Proc. EUROTRIB 85, Ecully, Elsevier, Amsterdam, Article 5-3.7, 10 p.
24.Petryk H. (1987). In Friction, lubrication and wear, pp. 987-994, Int. Conf. Tribology, London, I. Mech. E., C140/87,.
25.Black A. J., Kopalinsky E. M. and Oxley P. L. B. (1988). Wear 123,97.
26.Kailas S. V. and Biswas S. K. (1993). Wear 162-164, 110.
27.Samper V. and Felder E. (1990) In Mechanics of Coatings, pp. 271-279, Tribology Series 17, Dowson D., Taylor C. M., Godet M. (Eds) Elsevier Amsterdam.
28.Felder E. and Rouault de Co1igny P. (2004).pp. 203-210, Proc. 2nd International Conference on Tribology in Manufacturing Processes ICTMP 2004, Nyborg, Denmark.
Mechanical analysis of the scratching of metals and polvmers at moderate and large strains |
55 |
29.Bucaille J. L. (2001). Simulation numerique de /'indentation et de Ia rayure des verres organiques, These de Doctorat en Sciences et Genie des Materiaux, Ecole des Mines de Paris.
30.Ramond-Angelelis C. (1998). Analyse mecanique des essais d'indentation sur materiaux elasto-p/astiques homogenes ou multi-couches. Application aIa caracterisation de Ia
rheologie et de Ia tenue mecanique des films minces, These de Doctorat en Sciences et Genie des Materiaux, Ecole des Mines de Paris.
31.G'Sell C. (1995). In Introduction aIa mecanique des polymeres pp. 141-168, G'Sell C., Haudin J. M. (Eds) Institut National Polytechnique de Lorraine.
32.Bucaille J. L., Felder E. and Hochstetter G. (2002). J. Mater. Sci. 37, 3999.
33.Bucaille J. L., Felder E. and Hochstetter G. (2001 ). Wear 249, 422.
34.Storakers B. and Larsson P. L. (1994). J. Mech. Phys. Solids 42, W2, 307.
35.Goddard J. and Wilman H. (1962). Wear 5, 114.
36.Moore A. J. W. and MeG. Tegart J. (1952). Proc. R. Soc. Lond. A 212, 452.
37 Bucaille J. L., Felder E. and Hochstetter G. (2004). ASME J. Tribal. 126, 372.
38.Gane N. and Skinner J. (1973). Wear 24,207.
39.Boyce M. C. and Arruda E. M. (1990). Polym. Eng. Sci. 30, 1288.
40.Lucas B. N., Oliver W. C., Pharr G. M., Loubet J.-L. (1997). Mat. Res. Soc. Symp. Proc. 436,233.
41.Hochstetter G., Jimenez A. and Loubet J. L. (1996). J. Macromol. Sci .Phys. B38, N° 5- 6,681.
42.Adams M. J., Allan A., Briscoe B. J., Doyle P. J., Gorman D. M. and Johnson S. A. (2001). Wear 251, 1579.
43.Kermouche G., Barge M. and Bergheau J. M. (2003).In Assemblages: des materiaux a Ia structure, pp. 321-324, Actes du Colloque National MECAMAT, Aussois.
56
CHAPTER3
FRICTION, DAMAGE AND STICK-SLIP IN THE SCRATCHING OF POLYMERS
S.L.ZHANG
Department ofMechanical Engineering, University ofRochester, Rochester, NY 14627 Present address: Xerox Corporation, Bldg. JJJ-30N, 800 Phillips Road, Webster, NY 14580 E-mail: John.S.Zhang@xerox.com
ABSTRACT
This chapter presents results of friction, scratch morphology and stick-slip characteristics during the scratching of a selection of polymers (uncrosslinked and crosslinked poly(n-butyl acrylate) (PnBA) coatings, poly(dimethylsiloxane) (PDMS) coatings, and a bulk styrene-acrylonitrile (SAN) copolymer). The chapter describes in detail the dependence of the horizontal force or friction force on the normal load and driving speed, scratch damage and mechanism, critical normal load for scratch damage, and slip process of stick-slip motion. During the scratching of PnBA and PDMS coatings, the horizontal force appears proportional to the contact area that depends on the normal load, and the scratching is a rate process in which the viscoelastic property of the coatings influences their frictional behavior. Below a critical normal load, crosslinked PnBA and cured PDMS coatings recover elastically after being scratched so that there are no scratch marks left behind. The study on the slip process during the scratching of SAN copolymer indicates that during slip the scratch velocity starts from zero, increases to a maximum and then decreases to zero again. The scratch groove made during slip shows a nonuniform depth, which increases with the decrease of scratch velocity.
KEYWORDS
Scratching, friction, poly(n-butyl acrylate) (PnBA), poly(dimethylsiloxane) (PDMS), styreneacrylonitrile (SAN), coatings, elastomers, crossslinking, JKR theory, adhesion, damages, cracking, delamination, stick-slip, slip velocity.
INTRODUCTION
Polymer coatings have important tribological applications. Their friction and wear properties are of practical importance and of considerable scientific interest. There are many parameters that can be expected to affect the frictional properties of polymer coatings. However, this subject has not received sufficient attention to date [I].
Scratch testing is an important tool for the characterization of the tribological properties of materials. In this test, a hard object such as an indenter or a stylus is pressed onto the material surface under a normal load and moved by a horizontal force to produce a scratch in the